Additives and Ingredients
This section provides a list of common ingredients in paper products, along with descriptions of them. Click the "plus-box" to open up details and a graphic for each entry.
ACRYLAMIDE COPOLYMERS
Composition: This class of papermaking additives includes a wide range of molecular mass, degree of branching (often linear copolymers), and relative proportion of charged monomeric groups. By definition one of the monomers is plain acrylamide, i.e. -CH=C[-CONH2]- in which the group in brackets is attached to the second carbon. For production of an anionic copolymer, the co-monomer is often acrylic acid, -CH=C[-COOH]-. Cationic co-monomers typically have quaternary ammonium groups, and they are attached to the nitrogen of the amide, -CH=C[-CONH-RN+(CH3)3]-, where R can take a variety of forms. Polymerization is achieved by “zipping” the double bonds together – so the polymer backbone consists of single bonds (unsaturated). PAM copolymers used as retention aids have molecular masses in the range 2 to 15 million g/mole, and many of them are delivered as water-in-oil emulsions. PAM copolymers used as dry-strength resins are usually solution copolymers (no oil present) with molecular masses up to 1 million g/mole.
Functions: Retention aids (if molecular mass > 2 million g/mole); dry-strength resins (if molecular mass < 1 million g/mole); pitch-control agent (if highly cationic with molecular mass < 1 million g/mole); micro-polymer drainage aids (if highly anionic and very highly branched with a corona structure).
Strategies for Use: In their role as retention aids, acrylamide copolymers are added very late to the process, typically either right before or after the pressure screens. The pressure screens always degrade the molecular mass, hurting efficiency of retaining fine particles during paper formation. However, when the screens are used to deflocculate the fibers after the furnish has been treated with retention aid, then the resulting paper is more uniform. In their role as dry-strength agents the most critical aspect of acrylamide copolymer use is to balance the colloidal charge. Typically an oppositely charged material has to be added to the furnish either before or after a negatively charged strength agent. For control of pitch it is often recommended to treat the offending stream of thick-stock with just enough polymer so that filtrate from that stream has a low turbidity. Micro-polymers (see microparticles) usually are added last to the process, after a very-high-mass retention aid of opposite charge. The most critical factor is that the furnish have the same net colloidal charge as the retention aid at that point (usually by adding a charge scavenger).
Cautions: These materials can be very slippery when spilled. The emulsions of very-high-mass retention aids are particularly hazardous in this regard. Spills should be first wiped up with dry absorbent before rinsing.
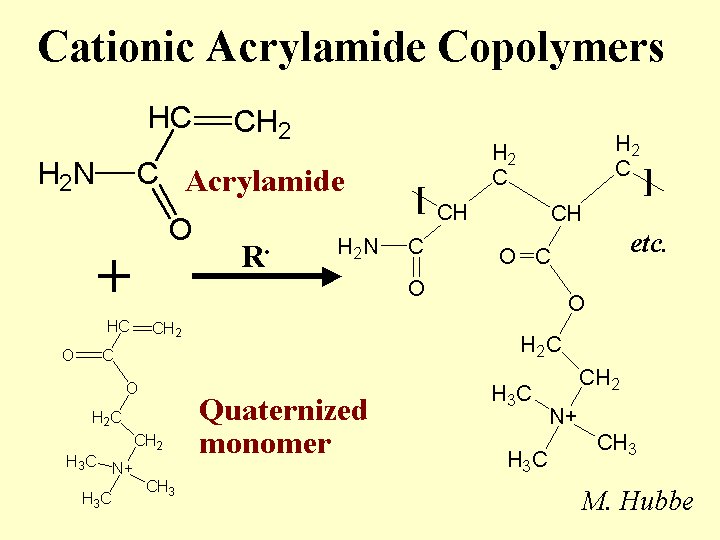
ALKENYLSUCCINIC ANHYDRIDE (ASA)
Composition: The active ingredient of ASA is an oily monomer. For purposes of papermaking, the most important components of this monomer are a five-membered anhydride ring and a linear chain having between 14 and 20 -CH2- groups (often 18). The reactive ring can be at various positions relative to the chain, and most commercial ASA consists of a mixture of these isomers. The product is almost always delivered as a light amber oil that must be kept very dry until emulsification. ASA is added to the furnish in the form of an aqueous emulsion, in which the stabilizer is usually cationic starch or another cationic, hydrophilic polyelectrolyte. Some suppliers also add a very small amount of surfactant to aid in emulsification. Likely contaminants include linear saturated oils, linear mono-unsaturated oils, and the hydrolysis products of ASA (see below).
Function: ASA is a sizing agent designed to increase resistance to water penetration in the case of paper formed under neutral or alkaline conditions. ASA is especially used in cases where full cure is desired before the size press and where it is important to maintain a high frictional coefficient in the paper product. ASA can improve paper machine runnability and preserve paper’s dimensional stability by limiting penetration of size-press solution into the sheet. Holding the size-press starch out nearer to the paper surface also can make the surface-applied additives more effective for such purposes as promoting surface strength, reducing dusting, reducing picking of vessel segments during offset printing, and even improving the performance of hydrophobic polyelectrolytes added with the size-press starch solution. Examples include styrene-maleic anhydride (SMA), styrene acrylates (SA), alkylated urethane copolymers, and certain emulsion products having chemistries related to SMA or SA.
Strategies for Use: The key goals in using ASA are (a) avoid hydrolysis, (b) distribute it well in the furnish, and (c) retain it efficiently. Hydrolysis is minimized by preparing the emulsion as late as possible – usually only seconds before the material is added to the thin stock. The cationic starch solution used in preparing the emulsion may be reduced in pH with the addition of such materials as adipic acid or alum, and it is usually cooled to some degree relative to its cooking temperature. A net ratio of about 3 to 5 parts cationic starch per part of ASA oil usually yields the most efficient sizing. To avoid excessive molecular chain cleavage of this starch, only a part of it is passed through the high shear zone of the emulsifier. Microscopic images (or other methods) can show whether one has achieved the desired narrow size distribution of droplets, usually with an average size near or below one micrometer. The recommended point of addition is after the hydrocyclone cleaners. Although the cationic starch sheath around each ASA droplet has some effect in attaching the size to cellulosic materials, a good retention aid system is needed to achieve a relatively high first-pass retention. Otherwise, a lot of the ASA will follow the white water circuit, giving it time to decompose. Deposit problems usually can be minimized by such practices as limiting the dosage (often to within 2.5 lb/ton in the case of virgin bleached kraft furnish), having alum or PAC present somewhere in the system, turning off the ASA flow during wet breaks, and maintaining good retention.
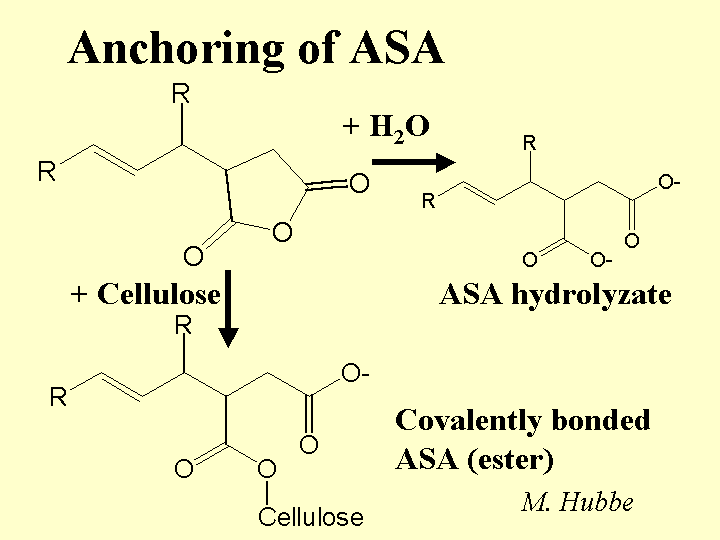
ALKYLKETENE DIMER (AKD)
Composition: This alkaline sizing agent is synthesized from fatty acids. The most common form is a waxy solid material dispersed as small particles in a solution that contains a stabilizer. The stabilizer may be cationic starch or another cationic polyelectrolyte. Recently the Hercules Corp. has patented the use of unsaturated fatty acids to make a liquid form of AKD. Though slightly less efficient as a sizing agent than the waxy form, the unsaturated product does not make the paper as slippery (see below). AKD is much less reactive than ASA, and there is no consensus between researchers as to how much of it ever forms covalent ester bonds with cellulose or starch when paper is dried.
Function: Hydrophobization of paper, especially when made under alkaline conditions. AKD is widely used for liquid containers, ink-jet printing papers, and many other grades of paper and paperboard. AKD is especially favored for products that need to resist water over a long period of time.
Strategies for Use: Because AKD is received at the paper mill as a ready-made, milky emulsion, it can be a very convenient product to use. Also, the lower reactivity of AKD, compared to ASA, means that the papermaker has more flexibility on where to add it. For example, many users of AKD add it to the thick stock; this practice tends to get the AKD to the fiber surfaces. In contrast, adding a sizing agent to the diluted furnish in the thin stock loop can be expected to concentrate more of the size onto the fines fraction. The presence of precipitated calcium carbonate (PCC) in the system can reduce the effectiveness of the sizing agent and also it may cause the sizing to lose some of its effect during storage (sizing reversion). Recent work reported by Bud Brungart of Hercules showed that this effect can be minimized by adding the PCC early in the process. The mechanism by which early addition of the PCC minimizes sizing reversion is not known, but it possibly involves coverage of the PCC by dissolved polymers and colloids from the pulp, dissipation of the surface alkalinity of the PCC, or increasing the bicarbonate content of the process water. Paper made with high levels of AKD is likely to be slippery, and it may cause problems in precision cutting and register during conversion, or in stacking during high-speed xerographic copying. These effects can be minimized by limiting the dosage (perhaps supplementing the sizing effect with surface hydrophobes added to the size-press starch), or by use of alkenylketene dimer (unsaturated form) in place of the more usual AKD.
Cautions: AKD hydrolyzes slowly during storage. Manufacturers instructions should be followed regarding the temperature and time of storage. Also consult the MSDS.
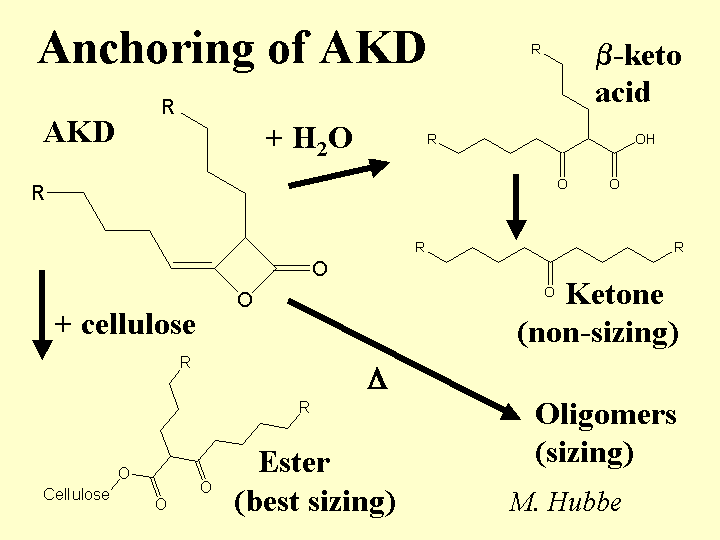
ALUM (Aluminum sulfate, or papermaker’s alum)
Composition: Papermaker’s alum is aluminum sulfate. Concentrations and addition amounts are usually based on either (a) the equivalent amount of Al2O3 or (b) the equivalent amount of the hydrate, Al2O3.14H2O. Alum is most commonly delivered as a liquid concentration, having a solids level of 8.3% as Al2O3 or about 50% as hydrate. Alum solutions are acidic. For instance, a 1% solution has a pH of around 3. Ionic species present in alum solutions are highly dependent on the degree of reaction with hydroxyl ions. It is tempting to say that “the composition is pH-dependent.” However, in the vicinity of pH=4.3 the composition of alum solutions changes a great deal with very little change in pH. At pH=3 and lower the main species is a hydrated form of Al3+. At a pH near to 4.3 there may be oligomers such as Al13O40H487+ or related species that contain sulfate. In a broad range of pH between about 6 and 9.5 the main species will be Al(OH)3 (and possibly related species that contain sulfate). The soluble aluminate ion predominates at pH values greater that 9.5.
Functions: Drainage enhancement, rosin sizing, part of certain retention aid programs, dye fixation, cationic source, acidic buffer. See also rosin soap size, rosin emulsion size.
Strategies for Use: A general discussion of alum use strategies has to start with the subject of pH. At pH values of about 4.5 and lower alum can be described as a “forgiving” additive. In other words, the user has a lot of flexibility with respect to where and how much alum to use for rosin sizing and drainage. Because of the affinity of the sulfate ion for alum ions, an overdose of alum is not expected to cause the net colloidal charge of a papermaking furnish to be strongly cationic. Usually alum is added after rosin soap size to “set” the size onto the fiber surfaces. When the water hardness is high some papermakers reverse the order in order to minimize formation of calcium abietate and related rosin salts that are tacky and not efficient at making the paper hydrophobic. The same “reverse” order of addition also is used often when papermakers use rosin emulsion size products. Since the emulsion size is used at a somewhat higher pH range of 4.5 to 5.5 (or higher in the case of pseudo-neutral and neutral sizing) it becomes more critical that the time between the addition points for alum and rosin be minimized (but there has to be good mixing in between the additions). Alum still can play a very effective role in alkaline papermaking, even though the equilibrium species are uncharged. Apparently the alum is able to rapidly complex with and neutralize carboxylate species in neutral or alkaline furnish before it has reached its equilibrium ionic composition. Careful dilution with clean water and good agitation are required to avoid deposit formation when alum is used above about pH 5.5.
Cautions: Alum is acidic. See details in MSDS.
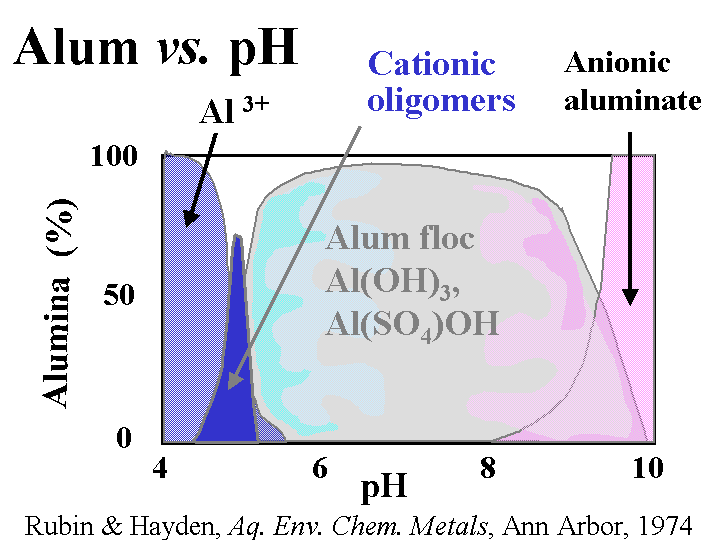
AMPHOTERIC STARCH
Composition: The word “amphoteric” implies that a chemical product has both positive and negative ion groups when it is dissolved in solution. When papermakers refer to amphoteric starches they are usually referring to products derived from corn starches. The least expensive starch products are based on dent corn, a corn variety that is also the source of most corn sweetener. Amphoteric starches also are prepared from waxy maize, a variety of corn that produces only the branched type of starch, amylopectin. The cationic groups are the same quaternary ammonium substituents used in preparation of cationic starches. The anionic groups usually are phosphates. Strictly speaking all cationic potato starches are really amphoteric starches since potato starch contains about 0.08% phosphorous. The cationic content of amphoteric starches is typically in the range of 0.2 to 0.3% nitrogen. All of these products are delivered to the mill as a dry powder having a moisture content of 10 to 20%.
Function: Enhancement of dry strength. Possibly useful for improving drainage and retention, especially in systems where alum is also used.
Strategies for Use: As in the case of other wet-end starches, the first step is “cooking.” The batch procedure takes about 20 to 30 minutes at about 95 oC at atmospheric pressure. Much more rapid cooking can be achieved in continuous “jet cooking” procedures at higher temperature and pressure. The performance of starch as a dry strength agent is only moderately affected by its point of addition. Addition to the thick stock has both potential advantages, and disadvantages. Advantages include getting a higher proportion onto long fibers, ensuring good retention and a relatively high effect on overall dry-strength. Also, addition to the thick stock ensures that any flocculation caused by the starch has been completely reversed before the headbox – making it possible to get a very uniform sheet (depending also on what is done with retention aids). Disadvantages of early addition include breakdown of starch by hydrodynamic shear and possible disappearance of some of the starch into the interior of pores in the fiber cell walls. For these reasons it is common to add at least part of the starch to the thin stock with only a few seconds of exposure to the furnish before it is made into paper. The performance of amphoteric starch can be enhanced if alum or polyaluminum chloride (PAC) is also being used. Amphoteric starches have a reputation for performing well under conditions of high electrical conductivity. Also, they can be an effective strength agent for systems that would tend to become overly cationic if a straight cationic starch was used.
Cautions: Starch products can be very slippery when spilled. Steam and steam lines in the vicinity of starch preparation areas can cause burns. The dry powder should not be breathed.
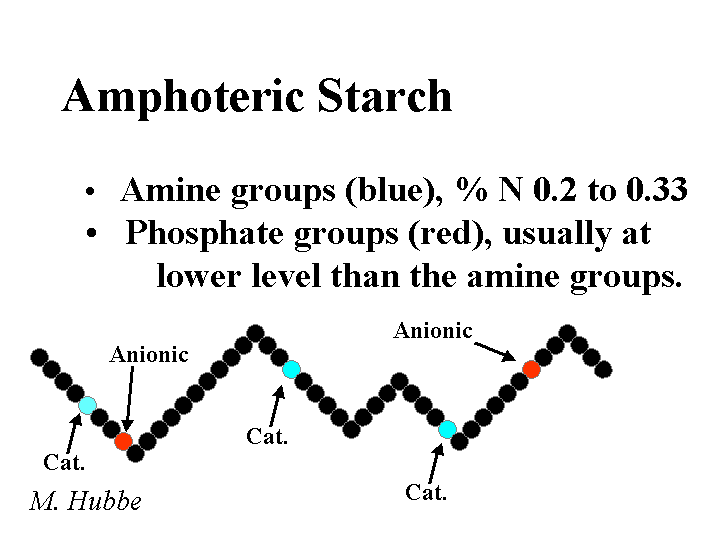
ANIONIC DISSOLVED AND COLLOIDAL SUBSTANCES
Composition: “Anionic trash” is one of the popular names used to describe a wide range of anionic dissolved polymeric and colloidal materials. Another common name is “DCS” for “dissolved and colloidal substances.” Many such substances come from the wood and get released during pulping. Some key components include fatty acid salts, resin acid salts, hemicellulose and its oxidation byproducts, lignin derivatives from kraft pulping, and dispersants or anionic latex and dispersants from coated broke. Various types of bleaching agents tend to oxidize hemicellulose and extractives, further increasing the anionic charge of the dissolved and colloidal fraction of the pulp slurry. Pulp washing of thickening stages after pulping and after major bleaching stages are intended to keep this material from going to the paper machine, but it is common for such devices to be working beyond their rated capacity.
Function: Anionic colloids and dissolved polymeric materials interfere with the performance of cationic retention aids, cationic dry-strength agents such as cationic starch, and wet-strength resins. Also they can play a role in deposit problems.
Strategies for Use: The best strategy for dealing with excess colloidal anionics is to employ highly efficient washing and keep these materials away from the paper machine. Some capital expenses for washing equipment can be justified in terms of chemical savings. That’s because another way to deal with anionic trash is to neutralize it. The most common chemicals for neutralization are alum (especially in the case of acidic papermaking), polyethyleneimine of polyaluminum chloride (PEI or PAC, for acidic or neutral systems), or polyamines or poly-DADMAC (for all pH ranges, but especially alkaline pH). The best strategy is to add the cationic material to the pulp stream(s) that is the major source. For example, it makes sense to add a cationic polymer to coated broke. Continuous evaluation of the colloidal charge by means of streaming current titrations makes it possible to keep the treatment level at an optimum point, even if the amount or quality of the broke changes. Other possible strategies include treatment of the process water with enzymes or biological digestion of the process water, i.e. secondary wastewater treatment and its reuse in the process. Because these cationic additives can increase the marginal cost of production, their use and optimum addition levels need to be justified in each application, based on demonstrated improvements in such things as retention, sizing, system cleanliness, or process stabilization, to name a few.
Cautions: In certain chemithermomechanical pulp operations with peroxide bleaching (BCTMP) and in certain neutral sulfite semichemical (NSSC) systems for making corrugating medium the level of anionic trash is so high that no one can afford to neutralize the system. Retention aids (except for PEO) and cationic starch, etc., do not perform well under such highly anionic conditions.
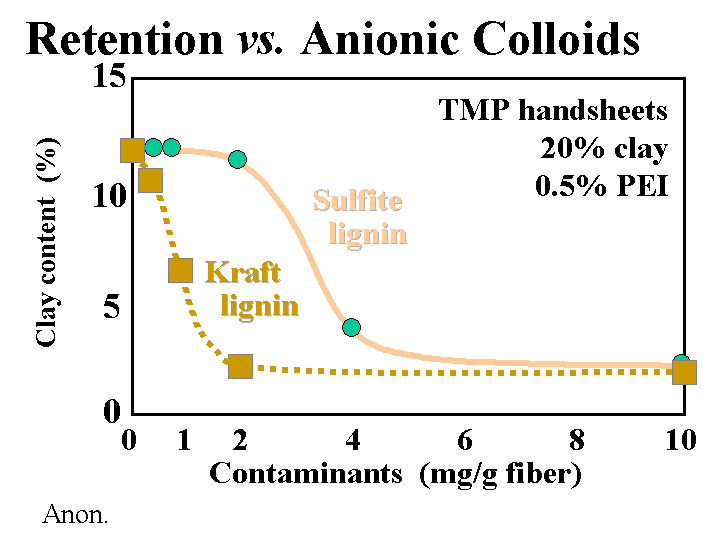
ANTI-FOAM / DEFOAMER
Composition: There is an extremely diverse set of chemical formulations that can be effective either to prevent foam (anti-foam) or to destroy it once it has formed (defoamer). Actually the distinction between these two terms is usually ignored. Most foam-fighting chemicals can serve either role. Let’s therefore use the term “defoamer” as a general label for all of these chemicals. The most universal characteristic of any defoamer is the fact that it is surface active, but highly insoluble in water. It has to be formulated so that it will be dispersed as tiny droplets, i.e. as an emulsion. The surface-active nature of the material causes it to spread very rapidly onto any air-water interface that it encounters. This is especially the case if that interface already is covered by the types of surface-active materials that tend to stabilize foams. Some, but not all defoamers contain hydrophobized silica particles or ethylene-bis-stearamide particles. The function of such particles is to pierce the surfaces of foam bubbles, causing them to coalesce when the defoamer spreads at the interface. In the past the main ingredient of many defoamers was oil. However, concerns about chlorinated organic products of bleaching have caused many pulpmakers and papermakers to switch to low-oil, 100% “active”, or silicone-based defoamers that don’t have this problem.
Function: To cause foam bubbles to coalesce to the point where they are large enough to float harmlessly to the water surface and break
Strategies for Use: The main concern is to use the correct dosage. Too little defoamer may mean that the paper machine does not achieve the required drainage performance, it may be very dirty, and there may be loss of materials as foam spills from the white water silo and other points in the process. Too much defoamer often adversely affects the performance of sizing agents, and dry strength agents, and it can cause or worsen deposit problems. The best approach is to test the air content of headbox furnish and cut the dosage to the minimum needed to keep air content at an acceptable level. Defoamer addition anywhere in the thin-stock loop will tend to eliminate air from the furnish in the white water silo and in the deculators (air-removing elements, if present). It is worth noting that even when bubbles are eliminated, some dissolved air will remain in the furnish, and this can be released as bubbles when the jet of stock leaves the high-pressure zone of the headbox.
Cautions: Any tacky deposits found in a paper mill are likely to contain defoamer materials. A large overdose of defoamer is likely to cause microscopic foam bubbles that can be very persistent.
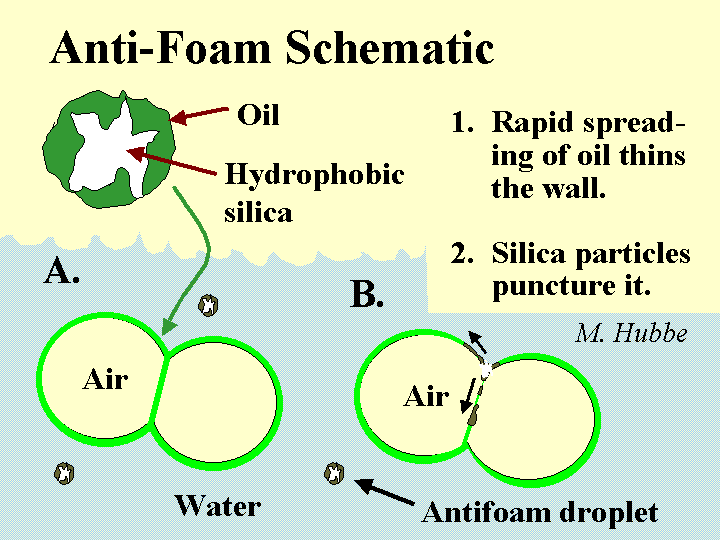
BENTONITE (sodium montorillonite)
Composition: Bentonite is a common name for the anionic smectite clay minerals used by papermakers as a retention and drainage additive or “microparticle.” Under the scanning electron microscope (SEM), bentonite particles can be almost indistinguishable from kaolin filler clay or coating clay. The main difference is the thickness. The sodium or potassium salts of bentonite exfoliate into extremely thin plates. In theory these plates can be as little as about 1 nm in thickness, yielding a huge surface area per unit mass. In practice, not all of the platelets will become separated from each other.
Functions: Retention and drainage (if added after or before a cationic retention aid); pitch control (if used in combination with highly charged cationic polymers).
Strategies for Use: First let us consider the role of bentonite as a retention and drainage promoter. Following the patented technology of Ciba Specialty Chemicals, bentonite added downstream of a cationic polyacrylamide retention aid causes a marked improvement in dewatering. Best results are achieved when sufficient very-high-mass cationic polymer (e.g. cationic acrylamide) has been added or is about to be added downstream so that the furnish momentarily has a net cationic charge. If the furnish has a high level of anionic dissolved and colloidal materials, then it makes sense to first treat the stock with a highly charged cationic material to neutralize most of this excess charge. The high aspect ratio of bentonite means that it has significant ability to bridge between different particles or fibers covered by the cationic polymer. That explains why bentonite tends to be very effective for retention, as well as for improving drainage. Patented technology from Vinings, Inc. shows that bentonite can be used in combination with highly cationic polymers for adsorption and detackification of pitch.
Cautions: When handling dry materials it is important to take proper precautions against airborne dust.
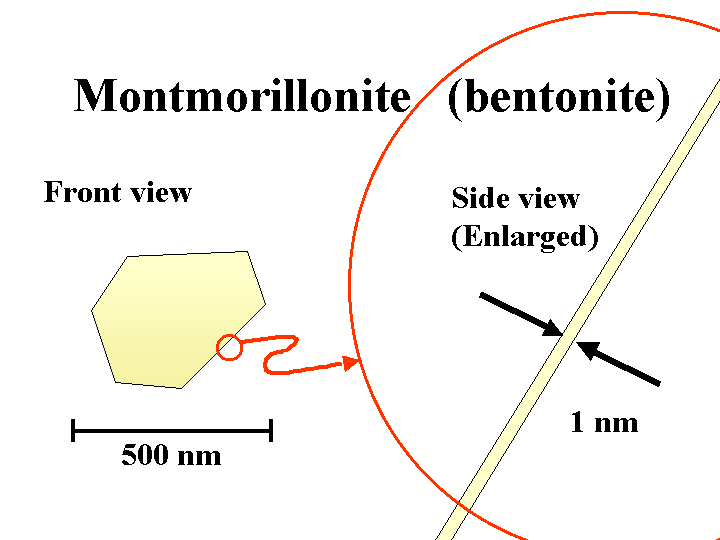
BIOCIDES
Composition: There are at least two main classes of biocide. On the one hand there are oxidizing agents such as chlorine dioxide and hydrogen peroxide. These happen to be the same chemicals that are also widely used for pulp bleaching. Therefore it is convenient for facilities employing these bleaching agents to add small amounts to the paper machine system as well. The oxidizing action either kills the bacteria and fungi outright, or it weakens the cell walls so that they are more susceptible to the other main class of biocides. The other class involves highly toxic organic chemicals. Subclasses of toxic biocides go by such names as thiazoles, thiocyanates, isothiazolins, cyanobutane, dithiocarbamate, thione, and bromo-compounds. As the names imply, many of them contain sulfur (“thio”-). A possible third category consists of materials that have an ability to inhibit biological film formation, e.g. surfactants such as alkylsulfosuccinates.
Function: One has to keep in mind that the main goal is to limit the growth of sessile bacteria, i.e. those that are attached to surfaces. These are the bacteria that tend to build up, cause slime deposits and holes, hurt productivity, and hurt product quality.
Strategies for Use: Grades composed of bleached pulps very often involve a combination of treatments with oxidizing biocides, supplemented by toxic organic biocides. The usual recommendation is to treat each of the incoming streams, including the fresh water, filler slurries, chemical additives and makedown streams, etc. Special attention has to be paid to the starch preparation area, since starch is such a favored food for slime growth. The level of oxidizing agent (e.g. chlorine dioxide) has to be maintained at a sufficiently low level that there are not any problems with bleaching of dyes or decomposition of starch, etc. A possible starting level to consider is a residual of 1 ppm of active oxidizing agent in the paper machine system. Hydrogen peroxide, when used as a biocide, is slower to act but longer lasting. As a consequence it needs to be controlled at a higher level of residual activity in the system. The toxic organic biocides can be selected based on the temperature of the system and on the relative needs to control either bacteria or fungal growth. It is common to cycle the toxic biocide on and off over periods from several minutes to several hours; by this means it is possible to reach a required threshold of activity and also minimize the cost of chemicals. Such practices of intermittent addition need to be checked to make sure that they do not cause excessive swings in first-pass retention or other problems. Certain biocides are known to contain anionic dispersants that interfere with retention. The residual level of oxidizing chemicals is most conveniently estimated by measuring the redox potential of the furnish with a Pt electrode relative to a standard reference electrode. The effectiveness of a biocide program is best evaluated with a combination of measurements, including petri-dish cultures of water, tests for the presence of biological deposits on surfaces, the slipperiness of wetted surfaces, and the level of smells within the facility.
Cautions: Biocides are probably the most toxic materials present in a paper mill and they need to be treated with great respect. Biocide addition systems should be installed and adjusted only by highly qualified and authorized people. Anaerobic conditions under deposits can cause release of hydrogen sulfide, which can build up in such locations as tanks. Never enter a tank until procedures are followed to make sure that the air is safe.
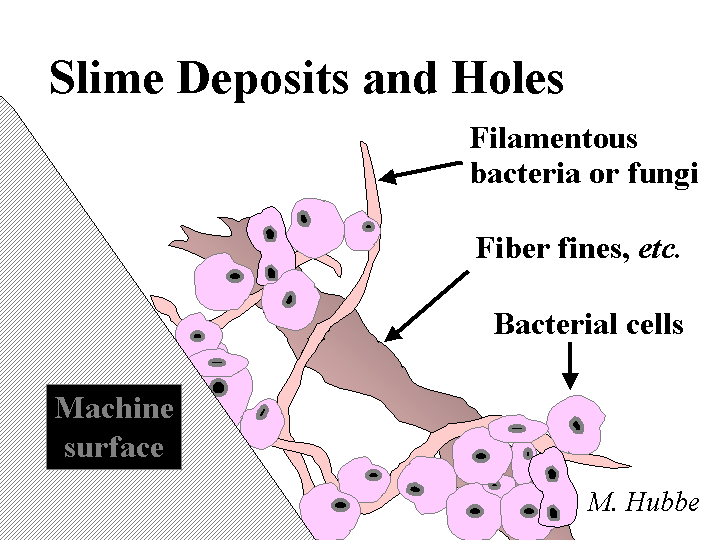
BLEACHED KRAFT PULP
Composition: Bleached kraft pulp consists mainly of cellulose and hemicellulose, two polymers that are composed of sugar molecules. The glucose units in each of these two chemicals are linked together in such a way (beta-1-4) that they are much more resistant to chemical hydrolysis than starch molecules. The cellulose chains have a great tendency to form crystalline domains that involve internal hydrogen bonding. These domains are responsible for the fact that the fibers do not dissolve in water. Hemicellulose has little tendency to crystallize due to the presence of side groups along the molecular chains. For this reason it functions like a glue, holding the fiber strands together and aiding in inter-fiber bonding when paper is produced. Kraft fibers are highly porous. The most important group of pores are slit-like and have a width between about 5 and 20 nm (based on a method called solute exclusion). Drying of the refined fibers causes some of these pores to close irreversibly and the fibers never regain all of their original flexibility and bonding ability when they are rewetted. Native cellulose fibers also have a central void area called a lumen. This void area usually collapses when the fibers are refined, formed into a sheet, pressed, and dried. Kraft fibers usually have a small amount of residual lignin and extractives (see pitch).
Function: Often the main component of paper, providing strength and other properties.
Strategies for Use: There is an economic incentive to use kraft pulp in the making of paper at the same site where it is originally produced. Such a practice avoids having to either (a) dry the fibers twice, or (b) ship large amounts of water. Also there is a loss of bonding ability each time that fibers are dried. Although this loss is moderate when drying unrefined kraft pulps, subsequent refining is likely to produce a greater level of fiber fines, and this will tend to slow the drainage. It is recommended to take care in the final washing of bleached kraft pulp to minimize carryover of highly anionic byproducts of oxidation. Market pulps are usually received as bales having a moisture content in the range of 10 to 20%, and these are dispersed with strong agitation in a hydropulper tank. Most grades of paper require the pulp to be refined by passing a slurry of 2 to 5% solids content between rotating metal bars of a refiner. The refining can be optimized by controlling the pH to the weakly alkaline range, controlling the energy content per mass of fibers (dry basis), and controlling the freeness of the resulting pulp. Excessive refining tends to hurt dewatering and bulk. By use of suitable dry-strength chemicals it is often possible to decrease the refining level and still achieve the specified strength levels.
Cautions: Pulp production involves a great many hazards. These include explosions of black-liquor recovery boilers, release of toxic gases such as chlorine and chlorine dioxide, and very high pressure steam. There are also many immediate hazards involved in handling of pulp bales with fork lifts, cutting of baling wires, and tossing bales into the maelstrom of a hydropulper.
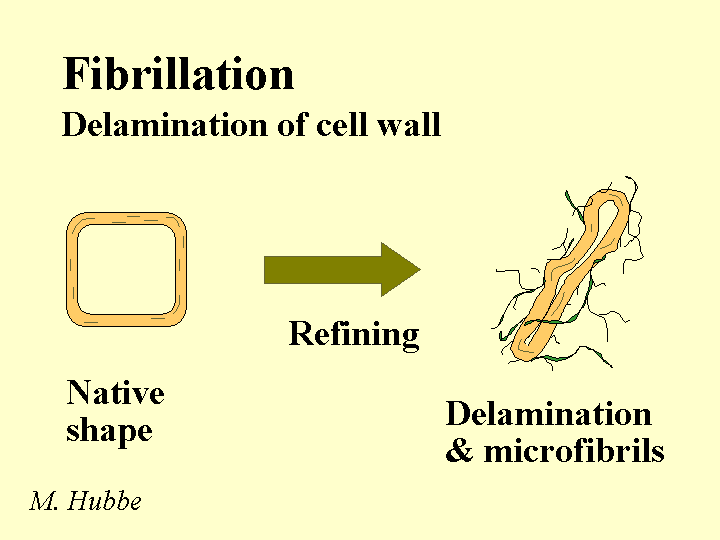
CALCIUM CARBONATE (filler)
Composition: The most important calcium carbonate products added to the wet end of paper machines have the calcite crystal form. In nature, calcium carbonate is abundantly provided in the form of limestone deposits, marble deposits, and chalk deposits. Of these three, the chalk deposits are by far the softest and most easily converted into suitable fillers. Chalk deposits form as a result of generations of marine organisms (coccoliths). This process results in particles that are rather more uniform, compared to products that result from simple grinding of isotropic solids. High quality chalk is abundant in England and in Denmark, but it is notably absent in North America. Limestone is much harder than chalk and very bright limestone deposits are found in such places as Alabama, Vermont, and British Columbia. Producers of filler-grade calcium carbonate have improved their ability over the years to narrow their particle size ranges and to eliminate large abrasive materials such as quartz sand. A much greater range of particle size, uniformity, and shape can be achieved by chemical means. Precipitated calcium carbonate (PCC) can be produced by “calcining” limestone at very high temperature to drive off CO2, “slaking” the resulting lime by addition of water, and then treating the resulting “milk of lime” suspension with CO2 gas under highly controlled conditions of temperature, pH, and ionic composition. The process results in calcite particles. Depending on the conditions of precipitation they may be either rosette (scalenohedral), blocky (rhombohedral), or various intermediate types. It is also possible to precipitate the aragonite crystalline form of calcium carbonate, and such particles tend to be needle-like (acicular).
Function: CaCO3 is used as a filler for making bright, opaque paper at minimum cost. Calcium carbonate also keeps the pH in the alkaline range (unless controlled with “acid-tolerant” technology). The rosette form of PCC filler tends to produce a bulkier sheet of paper; when calendered it yields improved smoothness at given levels of caliper.
Strategies for Use: Papermakers are most concerned that the filler content of the paper remain within tightly controlled limits. In particular, a momentary increase in the filler content may weaken the paper so that it either breaks or it is too weak to meet all of its specified strength parameters. On-line monitoring of mineral content (or “ash” content) is recommended. Incineration of samples of paper taken from the reel may be adequate for control of filler content in many cases. Considerations regarding the best point(s) of addition include (a) having an ability to rapidly adjust its content in the paper, and (b) avoiding interferences with certain other wet-end additives. In particular, calcium carbonate should never come into direct contact with acids, with alum, or with sizing agents. Acids cause the mineral to dissolve, releasing bubbles of CO2. This does not happen if the pH is kept above about 6.5. Alkaline sizing agents such as ASA and AKD work well under the aqueous conditions associated with calcium carbonate use, but they appear to have an adverse interaction with the mineral surfaces themselves. In particular, bonds formed between AKD and PCC during the drying of paper appear to be unstable during subsequent storage and use of the paper. The problem can be minimized by adding the PCC to the thick stock, well ahead of where the sizing agent is added.
Cautions: Standard precautions need to be followed. Calcium carbonate filler usually poses little danger to anyone. See the MSDS. Avoid generation of airborne dust.
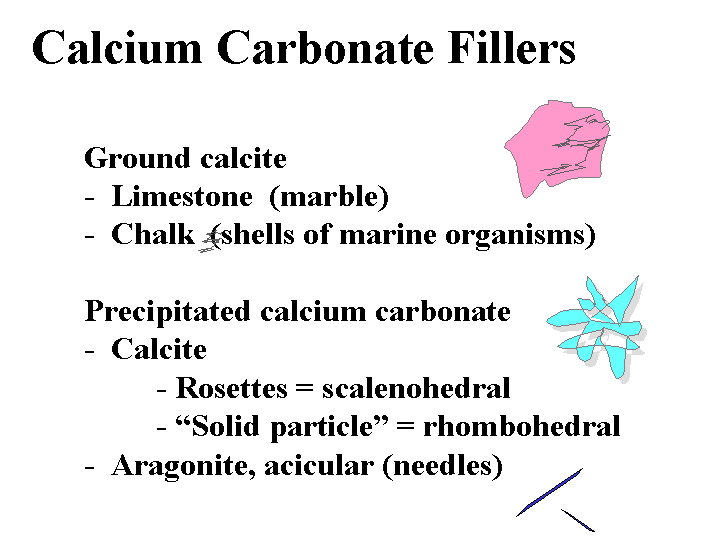
CATIONIC DIRECT DYES
Composition: Some common features of cationic direct dyes are (a) extended conjugation, (b) planar molecular structure, and (c) an excess of positively charged over negatively charged surface groups. In the Color Index scheme, cationic direct dyes are classified along with Basic Dyes. (Note: When papermakers refer to “basic dyes” they usually are referring to the products of somewhat lower molecular size that are commonly used in coloring mechanical fibers and other high-yield fibers. Such molecules are not considered to be “direct,” since they don’t have strong affinity to bleached kraft fibers.) The word “conjugation” implies an alternating series of double and single bonds, e.g. -CH=CH-CH=CH-CH=. In a direct dye molecule the chain of conjugation almost always contains two or more aromatic groups. Diazo groups, -N=N-, are also present in many dyes. The function of the extended conjugation is to achieve a suitably low energy for the promotion of the outermost electrons between the bonding and antibonding states, p to p*. Depending on its wavelength (or color), the energy supplied by a photon of visible light when it is absorbed may be able to promote an electron from the bonding to the antibonding state in the dye molecule. The color also can depend on substituent groups on the molecule that tend to supply or withdraw electron density. For this reason, ionic groups on a dye molecule can have a big effect on its color, and shifts in electrolyte concentrations can affect the hue. The word “direct” implies that the dye has a sufficient molecular size so that it has a strong preference to remain on surfaces. In other words, the user can add it “directly,” without a fixative or mordant, and it will stick to the surface. Cationic direct dyes have particularly high affinity for anionic surfaces due to their content of cationic amine groups.
Function: Coloration of paper, adjustment of the tint of white paper, and matching of a paper’s appearance to a standard agreed upon by the customer. Cationic direct dyes can be very valuable in cases where (a) the effluent water has to be colorless, or (b) where a high frequency of production changes between different colored products provides an incentive for keeping the white water free of residual dye.
Strategies for Use: The most notable issue in the use of cationic direct dyes is their tendency to “granite.” In other words, they tend to irreversible stain the first surfaces with which they come into contact. This can lead to a speckled appearance of the paper product. To avoid this, users must use adequate dilution and add the dye at a point of good agitation. Cationic direct dye never should be added to white water, since it will stain the unretained fines. Likewise, it should never be added in a chest, since it will stain the fibers that happen to be near to the point of addition. The second critical rule is to avoid direct contact between a cationic direct dye solution and streams of highly anionic additives. For example, a mixture of cationic direct dye and conventional (anionic) direct dye produces an insoluble tar. All direct dyes usually are delivered to a paper mill in the form of concentrates. These may be batch-diluted, though it is becoming more common to meter the concentrated dyes continuously with gear pumps or reciprocating positive displacement pumps. Rather than adding the concentrate directly to the process, the stream is combined with a relatively large amount of pure water (no fibers or fines). The dilution ratio is not critical. When producing deep shades of paper (highly colored) it is common to use a combination of cationic direct and (anionic) direct dyes, added at different points in the process. In such a situation the cationic dye can serve as a fixative for the anionic dyes, usually added later.
Cautions: See MSDS. Cationic direct dyes can cause objectionable and persistent stains of clothing (both yours and the paper machine’s).
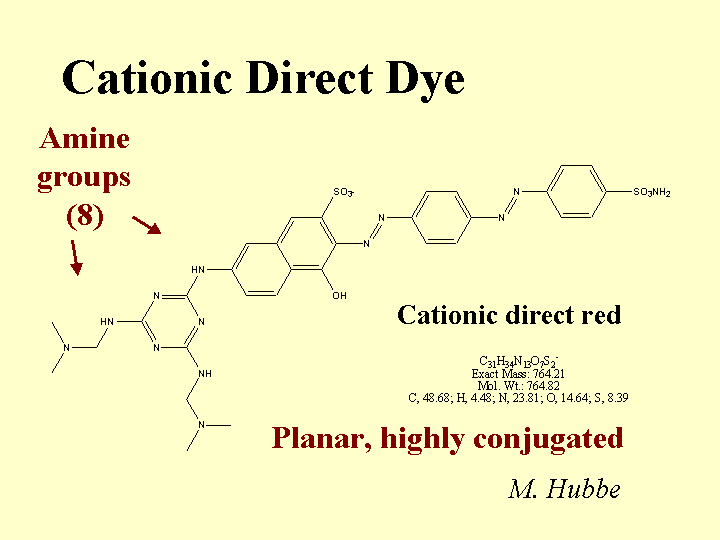
CATIONIC STARCH
Composition: The repeating unit of starch is glucose, having a carbohydrate monomer composition of C6H12O6. In the polymer each unit has three -OH groups, and the units are linked together with flexible alpha-1-4 glycocidic bonds. Cationic starch is produced by treating a slurry of partially swollen granules of starch with a reactive compound. An example of such a reagant is epoxypropyltrimethylammonium chloride. This reagant contains a quaternary nitrogen, yielding a positive charge that is independent of pH. The reagent usually attaches to the starch at the C6 position, the most accessible of the -OH groups. The typical level of derivatization is one to two charged groups per hundred glucose units. Because the reaction is usually carried out in a slurry, it is expected that the distribution of charged groups will be highly non-uniform. Also, there is reason to believe that treatment of potato starch or dent corn starch will result in preferential cationization of the linear amylose chains. The branched amylopectin chains of starch tend to be more crystalline in the solid starch granule, and therefore less accessible to treatments. Cationic starch is usually delivered in a dry powder form (10 to 20% moisture content).
Functions: Dry strength, emulsification of sizing agents, part of many retention and drainage programs
Strategies for Use: In the US cationic starch is the most popular dry-strength additive. About half the people who have an opinion will tell you that higher strength can be achieved by adding the starch to the thick-stock. The other half (of those who will tell you what they think) will advocate addition to the thin stock, i.e. later in the process. In either case you have to be a bit careful with the dosage. Depending on the furnish, the maximum practical amount of cationic starch may be between 10 and 30 lb/ton. The problem with adding too much is that it will exceed the adsorption capacity of the surface, based on either the surface area or the limited extent of negative charge of the surfaces of fibers and other solid surfaces in the furnish. Excess starch beyond what adheres to the fibers in likely to cause foam, high biological oxygen demand (BOD) levels in the effluent, and poor retention and drainage. The performance of cationic starch as a strength agent sometimes can be improved by raising the pH; this will tend to make the fibers slightly more anionic and better capable of interacting with the starch. If the furnish contains a very high level of anionic trash, then the performance of cationic starch as a strength agent can be improved by preteating the furnish with a highly charged cationic material such as alum, PAC, or polyamines, etc. Another strategy is to use a combination of cationic starch (first additive) and a microparticle such as colloidal silica or bentonite (second additive). Patents in these areas are held by Eka Chemicals, Nalco, and Ciba Specialty Chemicals, among others.
Cautions: Spills of cationic starch can be slippery. They should be cleaned up promptly with warm or hot water.
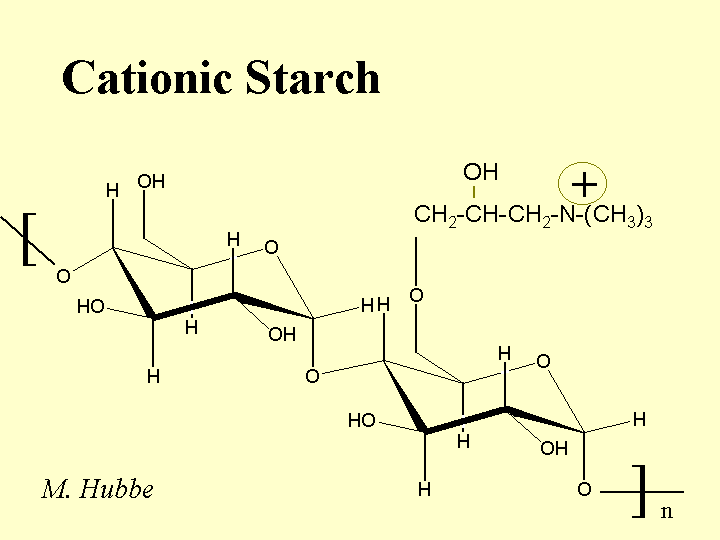
CHALK (a type of calcium carbonate)
Composition: Chalk is a form of calcium carbonate, having the same chemical composition as ground calcium carbonate, limestone, marble, and precipitated calcium carbonate (PCC). In fact, all of the calcium carbonates listed in the previous sentence have the same crystal form, calcite. What makes chalk different is its origin as the shells of little “coccolith” organisms. Generations of coccoliths gradually built up deposits. Since most of us have experience with chalk-board chalk it should come as no surprise that the deposited mineral is quite easy to grind. The particles released by grinding the chalk have a highly desirable property; almost all of them are in an excellent particle size range for use at relatively high levels as a filler. The moderately large size and low surface area (about 4 m2/g) relative to other common fillers means that chalk has less adverse effect on strength than most kinds of filler at any given filler content in paper. Especially abundant and high-quality chalk deposits are found in England and Denmark – but not in North America. This fact is probably part of the reason that the European paper industry was earlier in its widespread adoption of alkaline papermaking technology. The modest brightness of chalk (typically 80 to 86%) can be an issue, especially when it is compared against ground limestone and precipitated calcium carbonate products (both about 95 to 99% brightness). Also, chalk cannot be expected to achieve a high opacity. Opacity can be improved more by using something with a high surface area, bulking ability, and open structure, e.g. scalenohedral PCC. Chalk typically is dispersed with anionic materials such as phosphates or polyacrylates.
Function: Paper filler for cost reduction, pH buffering (alkaline), and superior ability to maintain acceptable paper strength as a function of increasing filler content.
Strategies for Use: Chalk filler is a relatively forgiving additive, as long as one avoids direct contact with certain other additives that can be adversely affected by it. These include acidic materials such as alum and sulfuric acid. Sizing agents typically should be well separated from calcium carbonate filler in the scheme of addition; usually the filler should be added early, and the size should be added late in the process. However, chalk is probably the most sizing-tolerant of all of the calcium carbonate filler varieties. Either a cationic or anionic retention aid can work well with chalk due to the presence of Ca2+ sites on its surface, and due to the presence of anionic materials (including filler dispersants) in the white water system. The amount of filler in the paper (if all of it can assumed to be chalk) can be determined by ashing the paper at 900 oC in a tared crucible, then dividing the “percent ash” by 0.56. This coefficient comes from the reaction of CaCO3 to CaO + CO2. In the presence of other minerals one needs to do a second ashing analysis for a standardized length of time at 500 oC or some other suitable temperature. Under such conditions almost all of the calcium carbonate remains as CaCO3.
Cautions: Since chalk is often delivered as dry powder, it is important to take precautions against breathing of the dust in the filler preparation area.
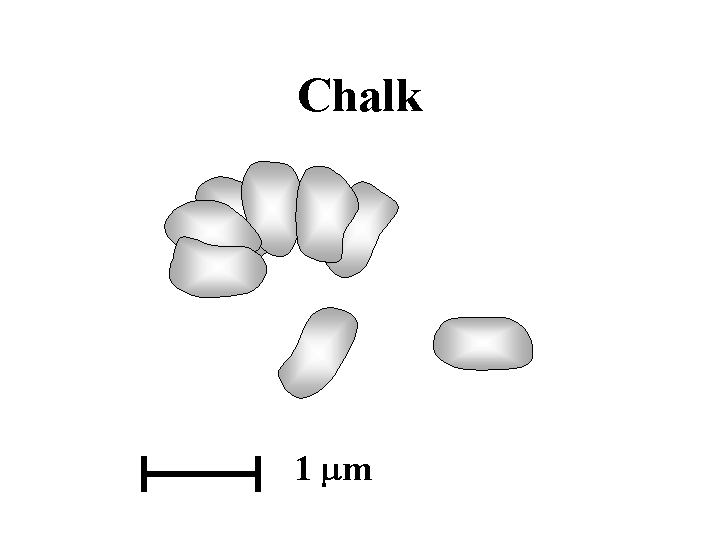
CHLORINE DIOXIDE (as a biocide)
Composition: ClO2. This is a reactive gas formed from chlorine and oxygen. It is a strong oxidizing agent with a tendency to open up unsaturated compounds and aromatic rings. In water solution it may be present as an ion with a single negative charge.
Function: Papermakers who use bleached kraft pulp are likely to have heard about chlorine dioxide even if they have never set foot in a pulp mill. That’s partly because it is very often present in the very last bleaching stage (D-stage), increasing the likelihood that some of it is carries over with the pulp into the paper machine system. Another reason is that it is one of the most popular biocide treatments for alkaline papermaking. Its use in that role is pretty much restricted to bleached grades, since any unbleached fibers will rapidly consume the chemical.
Strategies for Use: Chlorine dioxide has the desirable attribute of “quick kill” of bacteria and fungi, and it also decomposes relatively rapidly. It is conventional practice to treat the pulp furnish and then monitor the residual activity at a later point in the process. Typically the addition rate is controlled to give a residual chlorine content of about 1 ppm. The best residual level can be found by trial and error. Too much chlorine dioxide may adversely affect dyes, starch, and some other additives. Too little chlorine dioxide might result in a worsening of slime problems, bacterial counts, slippery surfaces, and odors. Excellent overall control of slime growth often can be achieved by combining chlorine dioxide treatment with the addition of organic chemical biocides.
Cautions: Generation of chlorine dioxide is hazardous and the equipment needs to be maintained by qualified people. The product is a very strong gaseous oxidizing agent.
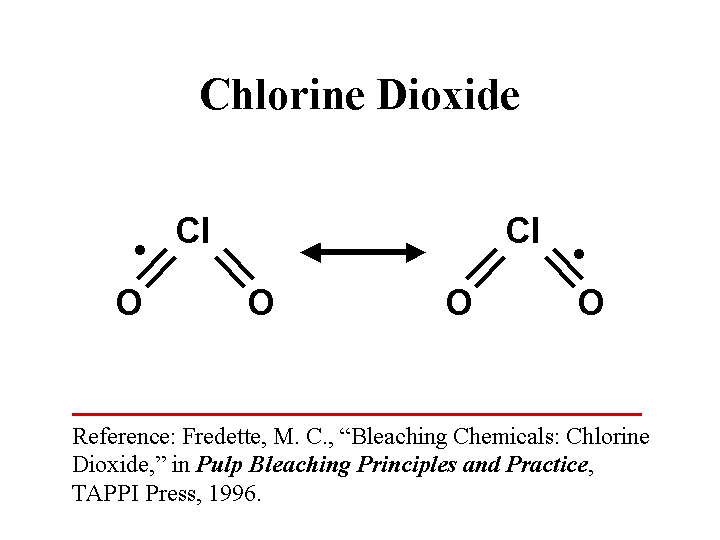
CLAY (filler)
Composition: This discussion will be limited to kaolin, the most common form of clay added to paper as a filler. (Bentonite, a generic term for montmorillonite clay, is a microparticle, and it is described elsewhere in this mini-encyclopedia.) Kaolin is a hydrated silica-aluminate. Deposits of kaolin particles have been built up by natural geologic processes. In one of these processes the kaolinite first becomes released by glaciation. Rivers carry silt in the glacial runoff and deposit it in shallow seas. Larger particles settle first, then the finer ones, usually at a different location. By choosing their locations, clay producers have access to a range of particle sizes and brightness of kaolin particles. High quality deposits of kaolin, having moderately high brightness and uniform particle size are found all over the world, but especially in China, Georgia, Brazil, and England. Anionic dispersants such as phosphates and acrylates are usually added to clay as it is collected and processed. The particles are typically irregular hexagons with a layered underlying structure. The size is highly variable; filler clays usually are selected with an average (SEM) particle size of about 1 to 3 micrometers. When the kaolin is first released it is likely to contain dark and abrasive impurities. Processing steps can include sieving, centrifugal cleaning, magnetic removal of dark contaminants, and bleaching. After these processes the particles of kaolin is still likely to resemble “books,” comprised of layers. This is the most common type of clay used for paper filling, especially when it is important to maintain strength at a given filler level. Extended agitation of clay slurries in the presence of hard ceramic balls (of visible size, much larger than the kaolin particles) causes the kaolin to delaminate into thinner platelets. This is delaminated clay.
Function: Besides reducing the cost of paper, clays are especially useful for creation of gloss (in highly filled, supercalendered papers), for increasing resistance to air flow (in the case of delaminated clays), and for imparting a moderate decrease in the friction coefficient of paper (especially when added to the size-press solution).
Strategies for Use: The main concerns are (a) how high a filler level is best, and (b) how to achieve an adequate retention. A uniform dispersion of clay can be obtained with conventional dispersing equipment, e.g. a Cowles mill. Also it is possible to get bulk delivery of clay slurries with solids levels in the neighborhood of 70%. The price that one pays for the convenience of using pre-slurried clay is that it contains anionic dispersants. These can make retention more difficult. Coating grades of clay are especially difficult to handle at high levels in the wet end due to the combination of dispersants, higher surface area, and smaller particles that are harder to filter from the water as the paper is being formed. The content of clay in paper usually is limited by decreasing strength properties and decreasing caliper at a given smoothness and basis weight. Clay tends to produce dense paper, especially when it is calendered. Unlike calcium carbonate fillers, clay products can be used at any pH. Clay will form agglomerates if prematurely mixed with such additives as cationic starch, alum, retention aids, and the like. Intentional agglomeration of filler is a potential way to increase the strength of paper at a given filler content; however this technology has not become widely used. Most retention aid systems are effective with clay. Ashing of the paper at 900 oC drives off waters of hydration. If a paper sheet is assumed to contain only hydrous kaolin (not calcined clay), then the percent ash needs to be divided by 0.86 to estimate the percent of clay originally present in the paper.
Cautions: Normal precautions regarding dust need to be observed when clay is received as a dry powder.
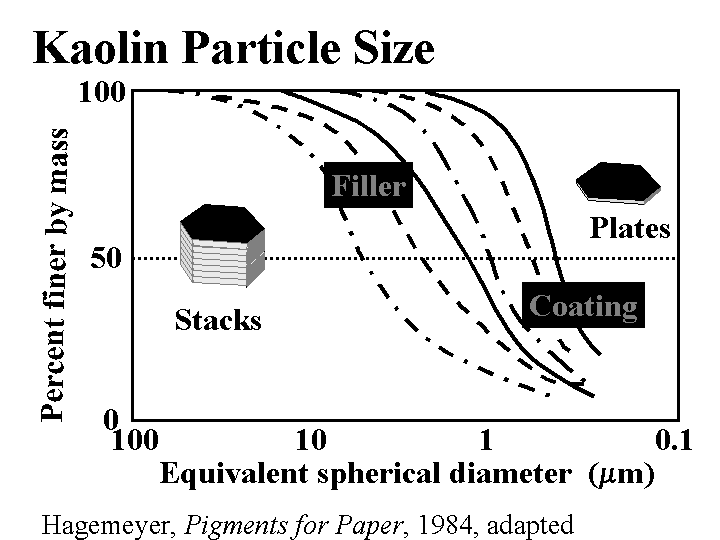
COLLOIDS
Composition: The word “colloids” says nothing about chemical composition. Rather, the word implies materials that have at least one dimension that is smaller than 1 micro-meters. Almost everything the papermakers deal with can be considered to be colloidal. Although fibers are larger than the classical definition, the fiber surface is highly porous, and microfibrils of colloidal dimensions extend out into solution from the surface of a refined cellulose fiber. Other colloidal particles common in papermaking furnish include fiber fines, filler particles, sizing emulsion particles, and retention aid molecules (i.e. molecules so big that they no longer behave like regular molecules). The average end-to-end distance of a retention aid polymer (e.g. 500 nm) is much larger than the size of a typical colloidal silica microparticle (e.g. 2 to 5 nm per subunit). When papermakers refer to colloids, they usually are most interested in the colloidal organic materials, including fatty acids, lignin byproducts, and oxidized hemicellulose. These are often called “DSC” for “dissolved and colloidal materials,” or “anionic trash.”
Function: By considering the colloidal properties of all of the materials that go into the wet end, it is possible to develop more efficient addition strategies and quality improvements in some cases.
Strategies for Use: Papermaking objectives that are most dependent on colloidal principles include deposit control, retention, drainage, and sizing. Let’s start with deposit control. Deposits form on papermaking equipment due to the thermodynamic instability of many materials suspended in water. Papermakers can combat this by getting those materials to deposit onto fibers, thereby keeping their concentration low in the liquid phase. Retention of colloidal materials is best achieved by a combination of coagulation (i.e. treatment to neutralize charges, causing the particles to come out of suspension) and flocculation (i.e. treatment with polyelectrolytes so large that they can bridge between the surfaces, even in the presence of net electrostatic repulsion between them). In contrast, exceptionally poor retention of fine materials can occur if the surfaces are coated with water-loving molecules with long loops and tails extending from their surfaces, i.e. steric stabilization. Drainage usually improves as the colloidal charge of the system is made closer to the neutral point and as fiber fines are retained to a greater degree onto the surfaces of longer fibers. Sizing requires that the water-loving (hydrophilic) surfaces of cellulosic materials be converted into water-hating (hydrophobic) surfaces. This requires treatment with molecules having hydrophobic groups, an ability to be well dispersed in the solution, an efficient mechanism of retention, and a means of becoming anchored and oriented at the fiber surface either during formation or when the paper is dried.
Cautions: Colloidal particles cannot be seen, except by viewing scattered light from a beam passing through the solution. Liquids that appear to be pure water might possibly be colloidal silica (which happens to be non-toxic, but the same cannot be assumed for all colloidal materials).
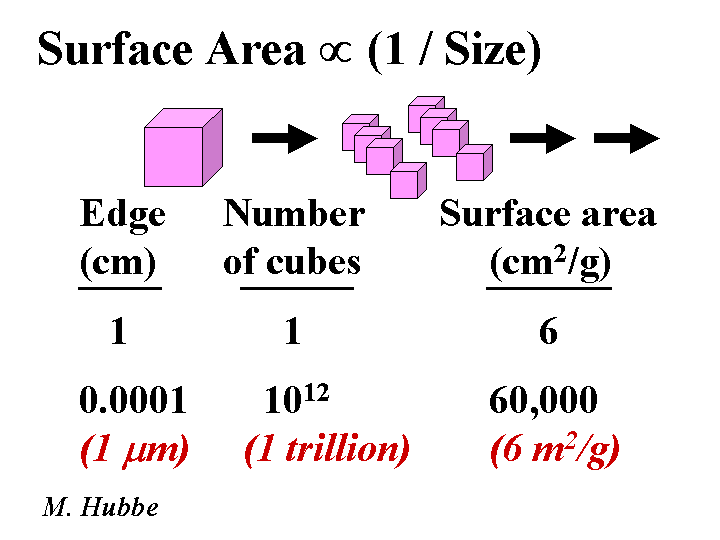
COLLOIDAL SILICA
Composition: Despite the fact that colloidal silica has the same chemical formula as quartz sand, SiO2, the two materials could hardly be more different in their effect on paper machine operations. The key difference is size. The subunits of colloidal silica particles are typically in the range of 1 to 5 nm. Whether or not these subunits are joined together depends on the conditions of polymerization. Initial acidification of a water-glass (sodium silicate) solution yields Si(OH)4. If the pH is reduced below 7 or if salt is added, then the units tend to fuse together in chains. These products are often called “silica gels.” If the pH is kept slightly on the alkaline side of neutral, then the subunits stay separated, and they gradually grow. These products are often called silica sols. Hydrogen ions from the surface of colloidal silica tend to dissociate in aqueous solution, yielding a high negative charge. Substitution of some of the Si atoms by Al is known increase the negative colloidal charge, especially when it is evaluated at pH below the neutral point. Because of the very small size, the surface area of colloidal silica is very high.
Functions: Key part of drainage-aid programs marketed by Eka Chemicals and Nalco; also capable of increasing the amount of cationic starch that can be retained as a dry-strength agent.
Strategies for Use: The conventional procedure is to add the colloidal silica very late in the approach flow to a paper machine, typically just after a set of pressure screens. A drainage rate increase is expected only if the furnish already has been treated with a suitable high-mass cationic polymer such as cationic starch or cationic poly-acrylamide. The effect is most pronounced when the net amount of cationic additives is enough to render the system at least slightly cationic before the addition of the micro-particle. For this reason it can be helpful to treat highly anionic furnish with a highly cationic material such as alum, poly-aluminum chloride (PAC), polyamine, or polyethyleneimine (PEI). In addition to scavenging excess anionic colloidal charge, such additives are expected also to make the molecules of the subsequently added cationic starch or cationic PAM adsorb with more loops and tails extending into solution. The function of the colloidal silica appears to involve (a) release of water from polyelectrolyte bridges, causing them to contract, and (b) acting as a link in bridges that involve macromolecules adsorbed on different fibers or fine particles. These effects create more streamlined paths for water to flow around the fibers. The tendency of microparticles to boost first-pass retention also will tend to have a positive effect on initial dewatering rates. It has been reported that paper produced by means of a microparticle retention and drainage program has a more open, porous structure, though the effect may become obscured by subsequent wet-pressing and calendering operations. Papermakers often are able to “trade away” chemical-induced drainage improvements in favor of improving formation uniformity. This is possible by either (a) decreasing the headbox solids by increasing the amount of white water recirculated, (b) increasing the proportion of hardwood fiber relative to softwood, or (c) increased refining.
Cautions: No significant hazards are reported. Read the MSDS.
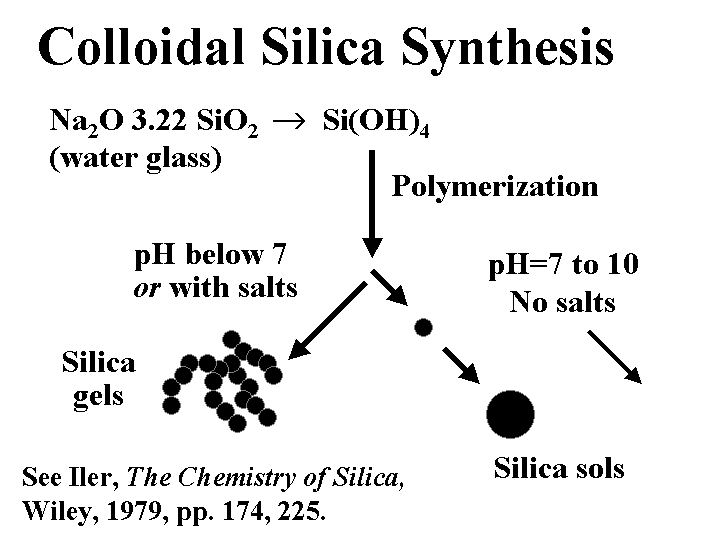
DEFOAMER (antifoam)
Composition: There is a diverse set of chemical formulations that can be effective either to prevent foam (anti-foam) or to destroy it once it has formed (defoamer). Actually the distinction between these two terms is usually ignored. Most foam-fighting chemicals can serve either role. Let’s therefore use the term “defoamer” as a general label for all of these chemicals. The most universal characteristic of any defoamer is the fact that it is surface active, but highly insoluble in water. It has to be formulated so that it will be dispersed as tiny droplets, i.e. as an emulsion. The surface-active nature of the material causes it to spread very rapidly onto any air-water interface that it encounters. This is especially the case if that interface already is covered by the types of surface-active materials that tend to stabilize foams. Some, but not all defoamers contain hydrophobized silica particles or ethylene-bis-stearamide particles. The function of such particles is to pierce the surfaces of foam bubbles, causing them to coalesce when the defoamer spreads at the interface. In the past the main ingredient of many defoamers was oil. However, concerns about chlorinated organic products of bleaching have caused many pulpmakers and papermakers to switch to low-oil, 100% “active”, or silicone-based defoamers that don’t have this problem.
Function: To cause foam bubbles to coalesce to the point where they are large enough to float harmlessly to the water surface and break
Strategies for Use: The main concern is to use the correct dosage. Too little defoamer may mean that the paper machine does not achieve the required drainage performance, it may be very dirty, and there may be loss of materials as foam spills from the white water silo and other points in the process. Too much defoamer often adversely affects the performance of sizing agents, and dry strength agents, and it can cause or worsen deposit problems. The best approach is to test the air content of headbox furnish and cut the dosage to the minimum needed to keep air content at an acceptable level. Defoamer addition anywhere in the thin-stock loop will tend to eliminate air from the furnish in the white water silo and in the deculators (air-removing elements, if present). It is worth noting that even when bubbles are eliminated, some dissolved air will remain in the furnish, and this can be released as bubbles when the jet of stock leaves the high-pressure zone of the headbox.
Cautions: Any tacky deposits found in a paper mill are likely to contain defoamer materials. A large overdose of defoamer is likely to cause microscopic foam bubbles that can be very persistent.
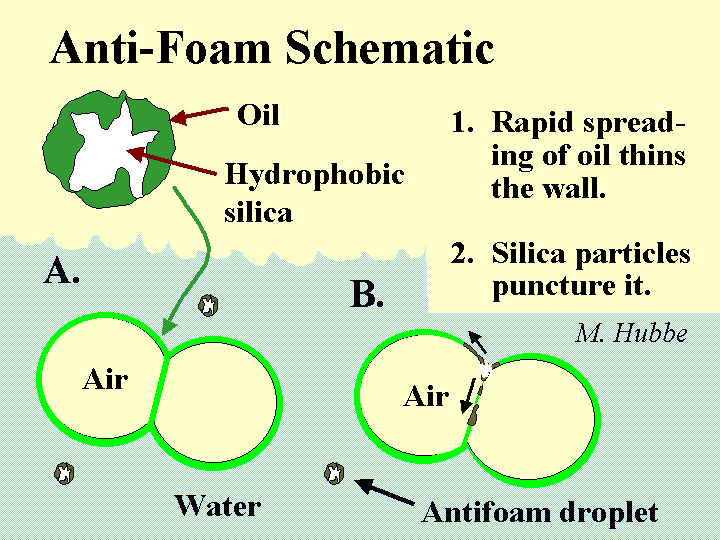
Mechanism of foam breakage by a defoamer. This involves spreading of an water-insoluble surfactant on the bubble surfaces.
DE-INKING ADDITIVES
Composition: Here we are mainly concerned with those additives or byproducts of de-inking operations that are likely to be carried over to the paper machine. Since there are a variety of different de-inking procedures, the nature of such carryover may be very different from case to case. De-inking operations that use flotation are likely to yield some carryover of fatty acid soaps or nonionic surfactants. De-inking operations that involve agglomeration of xerographic toner are likely to contain sparingly soluble nonionic surfactants. Operations that involve bleaching with hydrogen peroxide are likely to contain silicates. In addition, it is likely that the furnish will contain residual xerographic toner, which usually involves carbon-black pigment surrounded by thermoplastic resin binder.
Function: The goal of the papermaker is to use de-inked fiber to make paper that is essentially identical to the same grade of paper made from virgin fiber. The most challenging issues are dirt-counts, brightness, and strength.
Strategies for Use: The best strategies for handling of de-inked pulp all involve the de-inking plant, not the paper machine. Specialists in de-inking technology can provide guidance regarding combinations of screening, cleaning, agglomeration, dispersion, kneading, and floating that will give good efficiency, depending on the product requirements. An often-overlooked part of this process is the need to thicken or wash the final de-inked pulp so that soluble and colloidal material is not passed to the paper machine system. Once on the paper machine, contaminants and de-inking chemicals are likely to cause problems with production efficiency and quality. Addition of talc and other detackifiers to the wet end can help keep stickies in check. The dosage of sizing agent may have to be increased to compensate for the adverse effects of increased surfactant levels on development of hydrophobicity. Defoamers and biocides may have to be increased or changed when de-inked pulp is first introduced. The amount of de-inked pulp in certain fine paper grades may have a practical upper limit because of issues with brightness, cleanliness, friction, caliper, or strength.
Cautions: All recycling operations involve hazards due to the non-uniform nature of the material. Potential hazards include baling wires, hard objects, and toxic substances placed in the bales.
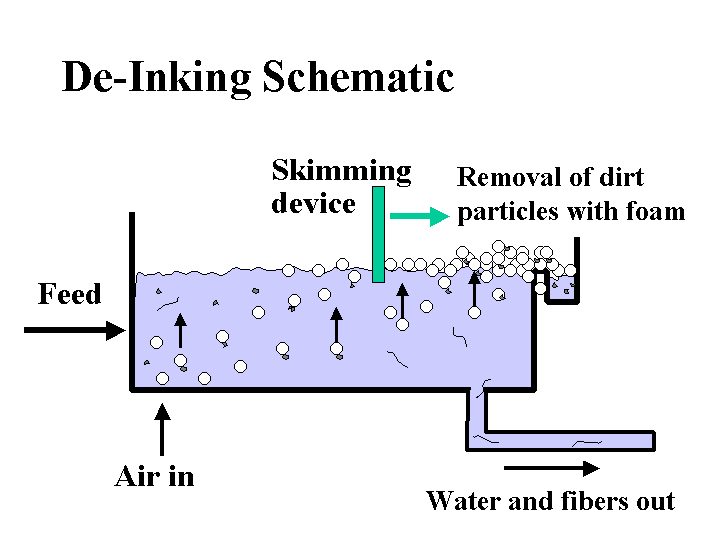
DETACKIFIERS
Composition: The most widely used detackifier is talc. The chemical formula of pure talc is magnesium silicate Mg3SiO4O10(OH)2. Talc is a soft mineral with a relatively water-hating surface. These two attributes, softness and hydrophobicity are easy to observe when one pinches some talcum powder and then releases it onto the surface of water in a sink. In addition to talc, there are a number of proprietary chemical products that also are marketed as detackifiers. The common feature of these chemicals is that they are weak polyelectrolytes (sometimes cationic), and they have a tendency to form a film at the surface of tacky or hydrophobic suspended matter.
Function: Reduce the tackiness of pitch-like materials or stickies so that they have less tendency to form agglomerates or deposit onto papermaking equipment or create spots in the product
Strategies for Use: Some of the key rules of thumb for talc are (a) disperse it well, (b) add it the those process streams that are giving the biggest problems, (c) add it early enough to the process with enough agitation, and (d) add enough. If too little talc is used, the talc is merely incorporated into deposits and agglomerates of tacky materials. It makes sense also to use an effective retention aid program in order to retain the talc (and any attached tacky materials), purging the problem from the wet-end system. Similar considerations apply to the use of organic detackifiers. In either case, the best place to start in dealing with deposit problems is chemical analysis. Several major papermaking research groups (including this one) have equipment for lab-scale simulation of pitch and stickies problems.
Cautions: Talc is a potential source of dust. Consult MSDS information for each detackifier. Lock-out procedures and air quality checks are required when tanks and headboxes must be entered to inspect deposits.
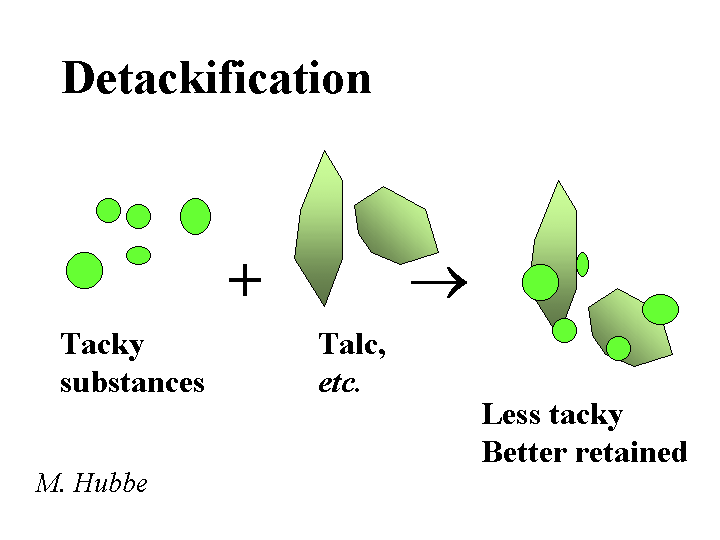
DIRECT DYES
Composition: The “direct dye” classification in the Color Index system refers to various planar, highly conjugated molecular structures that also contain one or more anionic sulfonate group. It is because of these sulfonate groups that the molecules are soluble in water. Though most direct dyes still can be obtained in powder form, it is increasingly popular to receive them as liquid concentrates. The advantage of concentrates is that they are easy to handle and meter. The disadvantage is that the surfactants and co-solvents needed to keep the dye concentrates stable may interfere with retention and sizing in the case of very deeply colored grades.
Functions: Coloration of paper, tint and shade control, correction of two-sided paper color.
Strategies for Use: Where you add the dye depends on such factors as the tintural strength of the desired paper color, the dye fixation strategy, and the color control strategy. Those responsible for process control will always advocate adding either all or some of the dye to the thin stock, within seconds upstream of where the stock comes out of the headbox. This practice can eliminate most of the time delay that is always a headache for online control strategies. But thin-stock addition might not give as high retention as addition to the thick stock. In some cases, especially in the past, it has been common to produce very deeply dyed products by adding the dye in a chest, giving the dye a long time to penetrate into the fiber cell walls. Other ways to achieve high retention of dyes include the use of highly cationic polyelectrolytes, alum, or polyaluminum chloride. The best efficiency of the colorant usually is achieved by adding the fixative ahead of the dye. However, the reverse order may give better rub-fastness or water-fastness. Another promising strategy is to add some cationic direct dye at a separate addition point upstream of the addition point from the (anionic) direct dye.
Cautions: The main hazards in handling of dyes are aesthetic; it is easy to stain hands and clothing. Consult the MSDS for other hazards.
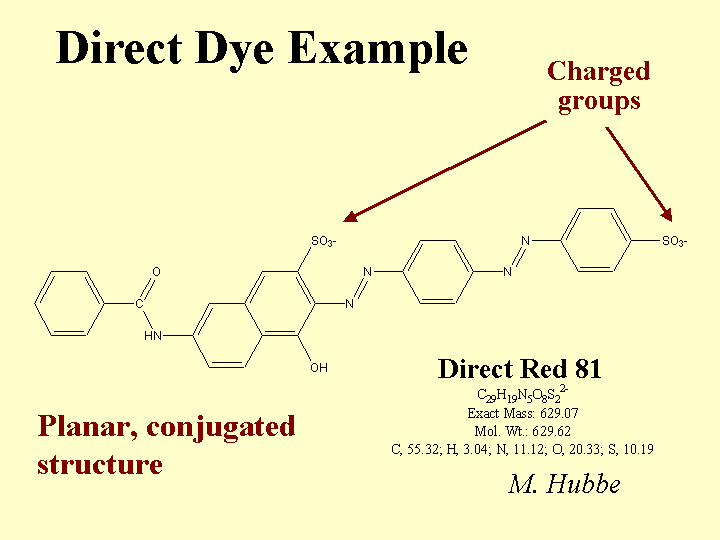
DISSOLVED AND COLLOIDAL SUBSTANCES
Composition: “Anionic trash” is one of the popular names used to describe a wide range of anionic dissolved polymeric and colloidal materials. Another common name is “DCS” for “dissolved and colloidal substances.” Many such substances come from the wood and get released during pulping. Some key components include fatty acid salts, resin acid salts, hemicellulose and its oxidation byproducts, lignin derivatives from kraft pulping, and dispersants or anionic latex and dispersants from coated broke. Various types of bleaching agents tend to oxidize hemicellulose and extractives, further increasing the anionic charge of the dissolved and colloidal fraction of the pulp slurry. Pulp washing of thickening stages after pulping and after major bleaching stages are intended to keep this material from going to the paper machine, but it is common for such devices to be working beyond their rated capacity.
Function: Anionic colloids and dissolved polymeric materials interfere with the performance of cationic retention aids, cationic dry-strength agents such as cationic starch, and wet-strength resins. Also they can play a role in deposit problems.
Strategies for Use: The best strategy for dealing with excess colloidal anionics is to employ highly efficient washing and keep these materials away from the paper machine. Some capital expenses for washing equipment can be justified in terms of chemical savings. That’s because another way to deal with anionic trash is to neutralize it. The most common chemicals for neutralization are alum (especially in the case of acidic papermaking), polyethyleneimine of polyaluminum chloride (PEI or PAC, for acidic or neutral systems), or polyamines or poly-DADMAC (for all pH ranges, but especially alkaline pH). The best strategy is to add the cationic material to the pulp stream(s) that is the major source. For example, it makes sense to add a cationic polymer to coated broke. Continuous evaluation of the colloidal charge by means of streaming current titrations makes it possible to keep the treatment level at an optimum point, even if the amount or quality of the broke changes. Other possible strategies include treatment of the process water with enzymes or biological digestion of the process water, i.e. secondary wastewater treatment and its reuse in the process. Because these cationic additives can increase the marginal cost of production, their use and optimum addition levels need to be justified in each application, based on demonstrated improvements in such things as retention, sizing, system cleanliness, or process stabilization, to name a few.
Cautions: In certain chemithermomechanical pulp operations with peroxide bleaching (BCTMP) and in certain neutral sulfite semichemical (NSSC) systems for making corrugating medium the level of anionic trash is so high that no one can afford to neutralize the system. Retention aids (except for PEO) and cationic starch, etc., do not perform well under such highly anionic conditions.
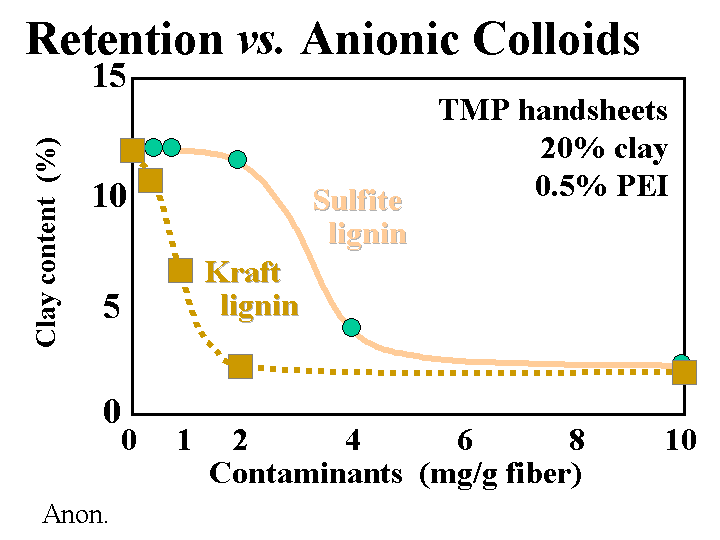
DRY-STRENGTH ADDITIVES
Composition: The term “dry strength additives” refers to a variety of water-soluble polyelectrolytes. The term “dry strength resin” is most often used in connection with anionic copolymers of acrylamide. Acrylamide polymers, including amphoteric products (with both anionic and cationic groups), are commonly used in Japan. Synthetic dry-strength agents tend to have molecular mass values below one million grams per mole. (Note that polyacrylamide flocculants used as retention aids often have much higher masses and this allows them to bridge between particles in suspension.) In the US it is far more common in North America for papermakers to use cationic starch. Other derivatives of natural products that are useful as dry-strength agents include carboxymethyl cellulose and guar gum derivatives.
Function: To increase the relative bonded area or strength per unit of bonded area between the fibers in a sheet of paper. Properties most likely to be favorably affected include internal bond strength and tensile strength.
Strategies for Use: Some keys to achieving the best performance from dry-strength additives include (a) getting it to retain onto the long-fiber portion of the furnish, (b) adding it to the thin stock (after the fan pump) so that it doesn’t have time to disappear into pores in the fiber cell walls, (c) avoiding conditions that tend to hurt the uniformity of formation, and (d) achieving a near-neutral colloidal charge. What is remarkable about this list is how many of the items appear to conflict with each other. For example, one might try to achieve item (a) by adding the polyelectrolyte to the thick stock, but such practices are in conflict with item (b) where one tries to minimize the time of contact with the furnish. Likewise, adding polyelectrolytes very late in the process (item b) is likely to increase the level of fiber flocculation, a conflict with item (c). Charge neutralization (item d) is clearly an excellent way to maximize retention of dry-strength additives but not all charge neutralization strategies will get the dry strength agent where it is most needed (item a). The costs and benefits of dry-strength additives always should be compared with optimization of refining conditions. Often the chemical approach will make it possible to achieve strength goals while maintaining somewhat higher dewatering rates and lower density of the paper.
Cautions: Consult MSDS information.
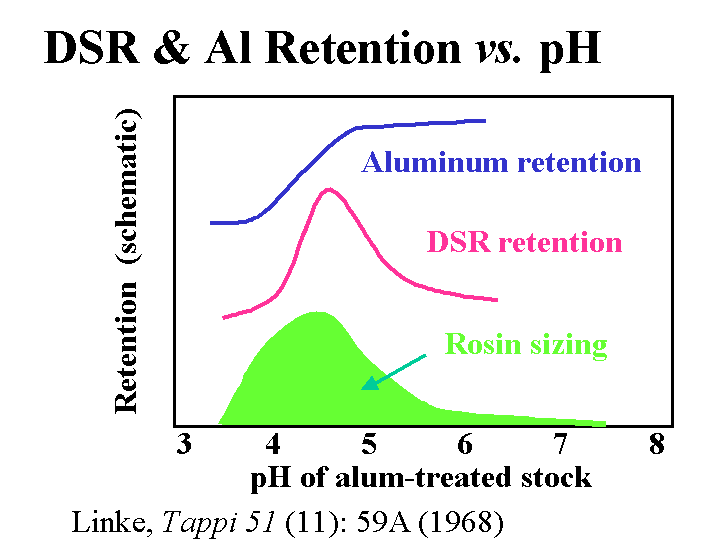
Effect of pH on the retention of anionic acrylamide copolymer dry-strength resin, sizing, and alum retention
DYES
Composition: Dyes, as used by papermakers, are grouped into five main categories. Two of these categories are so important that they have separate listings in this document. Direct dyes are by far the most widely used colorants for paper. They are noted for having a highly conjugated, planar structure, and an anionic charge due to the presence of sulfonate groups. The word “direct” implies that the molecule has enough tendency to stick to surfaces that no fixative is required. This is true for many, but not all dyes classified as “direct.” Cationic direct dyes are similar, but they have amine groups, providing a net positive charge. The combination of positive charge and relatively large, planar shape means that cationic direct dyes stick like crazy. Dyes classed as basic dyes also have a positive charge, but the molecules are not a large. They are used in highly anionic furnishes such as those prepared from mechanical pulp. Acid dyes, which have anionic charge and relative small size, are no longer very important in papermaking, due to their relatively poor affinity to fibers. Finally, insoluble colored pigments can be used in circumstances where high light-fastness is required. These have to be retained in the sheet with the help of a retention aid program, just like filler particles.
Function: Coloration of paper, shade control, white tinting.
Strategies for Use: Step one is to select suitable dyes for the grade and shade to be produced. The decision involves a process of elimination. Let’s assume that the goal is to match an existing green product for which the standard has been approved by a customer. Simple color-matching is easy – if all we are trying to do is to match the color under one standard condition of illumination. But real-life customers want more; they want the color to match the standard under all types of illuminant, everything from incandescent, to fluorescent, to sunlight. Experience has shown that such complete color matching can be achieved only if the hue of each major dye in the formulation matches the hue of a dye that was used in production of the standard. The field of worthy candidate dyes then can be narrowed by choosing to focus on the class or classes of dye (see above) most suited for the type of furnish. Some of the remaining dyes in the group of candidates may have to be rejected at this point because of grade-specific or process-specific requirements for bleed-fastness, light-fastness, bleachability, or affinity for different components in the furnish. Some dyes become known as “top-sided” dyes due to their tendency to adhere to filler and fiber fines – materials that tend to be enriched near the upper surface of a Fourdrinier sheet. By contrast, “bottom-sided” dyes achieve a greater depth of shade on the fiber-rich wire-side of a Fourdrinier sheet. Regardless of dye type, it is highly recommended to dilute it well.
Cautions: Dyes can stain hands and clothing.
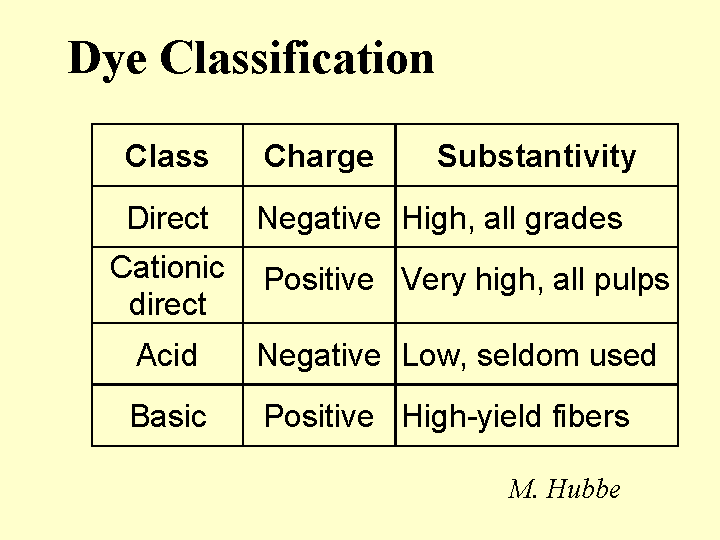
FIBERS
Composition: Since they contribute the majority of dry mass to a paper sheet, it is worth spending some time to describe them. The first issue involves the source of fiber. Most of the fibers from conifers (softwood trachieds) are about 3 millimeters in length and about 20 to 35 micrometers in width. By contrast libriform fibers from hardwood (deciduous) trees are typically about 1 mm in length and not as thick. Within these classes there is wide variety. Also there are effects due to the age of the tree, the environment, the season in which a particular fiber grows, the presence of shade, etc. Wood from deciduous trees is, in fact, a complicated mixture of these fibers, multicellular vessels (for transport of water from the roots), and other specialized cells. The next point of differentiation between papermaking fibers is the means by which the fibers are liberated from the wood. The kraft pulping method chemically dissolves the lignin, a natural phenolic resin that glues fibers together in wood. Kraft pulping yields fibers that tend to have superior bonding ability after they have been made flexible by the compression and shearing action of a refiner. The down side is that kraft pulping may remove more that half of the original mass of wood and some of the bonding ability and strength is lost when the fibers are recycled. By contrast mechanical pulps retain most of the original dry content, including lignin. Because lignin is stiff, these pulps behave differently. Also, the high content of lignin and extractable materials such as fatty acids and resin acids contributes to a higher density of anionic surface charge of mechanical fibers, compared to most kraft pulps.
Function: Fibers form the main structure of a sheet of paper and their nature determines the potential for strength development, opacity, density, and porosity, etc.
Strategies for Use: Papermakers are well aware that different blends of fiber types make sense for different grades of paper. If one wishes to manufacture paper bags it would be foolish to consider using hardwood pulps; they simply would not have enough resistance to tearing. On the other hand, if one wishes to make fine writing papers, it would be foolish to consider 100% of the relatively large southern U.S. softwood fibers. The sheet would be too rough and too porous. But wait – if one were to use no softwood fiber at all in making that same writing grade, then it is quite likely that the web would be too weak to run efficiently on your paper machine. One of the most interesting cases to consider is that of the common corrugated box. The flat layers (the “linerboard”) are made from unbleached softwood kraft pulp to maximize resistance to edge-wide compression failure. However the interior corrugated or fluted part (the “medium”) is usually made out of high-yield hardwood. The relative high content of lignin in such fibers means that they will be stiff enough to play their essential role in keeping the two liners spaced at an optimum distance for strength. When two fiber types are to be blended, it is often the case that the best overall mix of properties can be achieved by separate refining of each type. For example, most softwood pulp can benefit from much greater refining energy input, compared to hardwood. Over-refining tends to reduce fiber strength and produce fines that impede dewatering from the paper.
Cautions: Each facility needs to develop safe practices that minimize the chance of accidents. Potential hazards involving pulp are too diverse to be listed here.
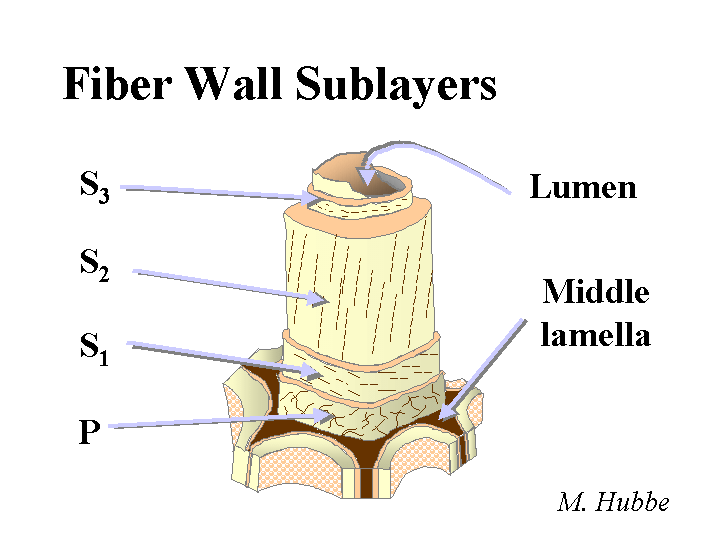
FINES (fiber fines)
Composition: When papermakers say the word “fines” they may be talking about just “fiber fines,” or they may be referring to everything solid in the furnish that is small enough to pass through a screen. Though researchers tend to define “fines” in terms of a standard sieve size, papermakers usually will consider something to be a fine if it can pass through the forming fabric used on that machine. Sometimes the term “fine particles” is used to make it clear that one is including such items of clay or calcium carbonate filler within the description, in addition to the fiber fines. Fines from wood fall into a couple of different categories. So-called “primary fines” consist of such items as parenchyma cells that store food in a living tree or transport liquids and foods in a radial direction. These tend to contribute bulk and some opacity to a sheet of paper. So-called “secondary fines” are produced by refining. Because secondary fines mainly are produced by delaminating the outer layers of the fiber, they tend to be slender and flexible – ideal for bonding. Unfortunately, these same fines, if the paper is recycled and then made into a recycled sheet, loose much of their bonding ability. Various studies have shown that fiber fines tend to have 3 to 5 or more times as much surface area per unit mass, compared to the fibers.
Function: Fiber fines, in general terms, tend to contribute to the smoothness and resistance to air penetration through paper. A moderate content of fines, e.g. up to about 10 to 35%, often achieves maximum strength, depending on the type of furnish and the quality of the fines. Fiber fines tend to impede dewatering throughout the papermaking process.
Strategies for Use: When they get desperate, papermakers occasionally will sewer their fines. But, when they can, they would much rather add the weight of the fines to what they sell to their customers. It often comes down to a question of finding a way to retain the fines well, without hurting the uniformity of the sheet. And it comes down to a way of overcoming the drainage problems associated with high fines levels. Conventional drainage aid strategies, retention aid programs, and micro-particle programs can be very effective. The conventional drainage aid programs appear to work by neutralizing the colloid charges at surfaces. Such neutralization tends to reduce the level of swelling of materials at fiber surfaces, and it also tends to make fibers at the fiber surface lie down flat on the surface. Retention aid treatment can help by binding the fines to fibers. The bound fines are prevented from following flow channels through the wet sheet of paper and becoming stuck at points of flow restriction within the structure. Micro-particle programs (involving prior treatment with a very-high mass retention aid) tend to achieve a combination of retention and charge neutralization, and so they can be beneficial when running a system containing a lot of fines.
Cautions: Ordinary safe procedures should be followed.
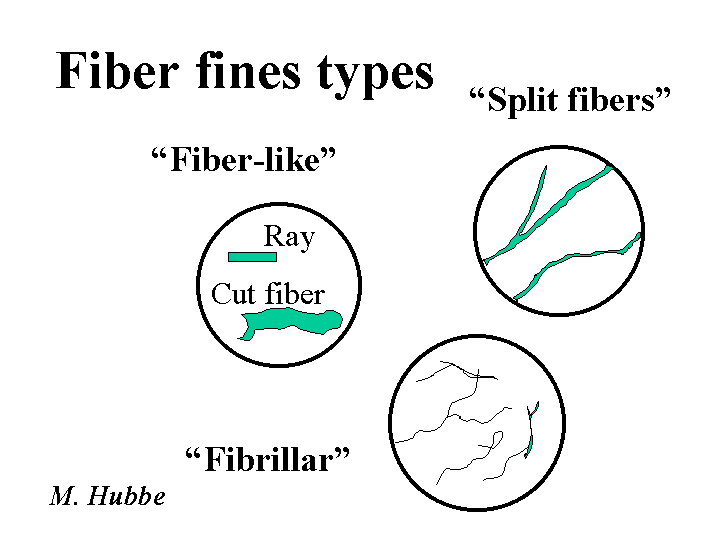
FILLER
Composition: When papermakers use the word “filler” they are talking about finely divided mineral products, usually in the size range of about 0.5 to 5 micro-meters. The most important of them are calcium carbonate and kaolin clay. Sometimes people also include titanium dioxide in the category of “filler” even though the particles tend to be smaller (e.g. 200-300 nm) and the term “filler” does not seem sufficiently dignified for a material that is so expensive. Some papermakers in places such as Asia and Finland regularly use talc filler having average particle sizes larger than 5 mm; this is possible because talc happens to be very soft and non-abrasive. Most fillers can be received by paper mills either as dry powders or as slurries. Precipitated calcium carbonate (PCC) is often prepared at a plant adjacent to the paper mill.
Function: Reduction of materials cost per unit mass of paper, increased opacity, increased smoothness.
Strategies for Use: The type of paper to be produced will have a huge bearing on the choice of filler, blends of fillers, and their level in the product. One thing to keep in mind is that fillers tend to interfere with inter-fiber bonding, reducing the strength of paper. The debonding effect of fillers is closely related to the mechanism by which they can contribute to brightness and opacity. The reason is that the fillers increase the amount of air-solid interfaces within the paper. Rays of light passing through paper are refracted or scattered only when they encounter a change in refractive index, such as occurs at air-solid interfaces. The most general rules for use of fillers include (a) making sure that the material is fully dispersed into individual particles before it is added, (b) mixing it with the furnish at a location that doesn’t adversely affect other additives, and (c) retaining it. The first goal and the last sometimes can be in conflict with each other, especially if a large amount of dispersant has been used to create a stable suspension. The most serious adverse interactions to avoid (item “b”) include mixing calcium carbonate with acidic materials, and allowing any coagulant or flocculant to contact the filler slurry before it is mixed with pulp. Various groups have explored the possibility of intentionally agglomerating fillers to improve paper strength. These strategies often seem promising, but they may be difficult to control.
Cautions: Dry filler materials are a potential source of dust; appropriate equipment has to be used to disperse them.
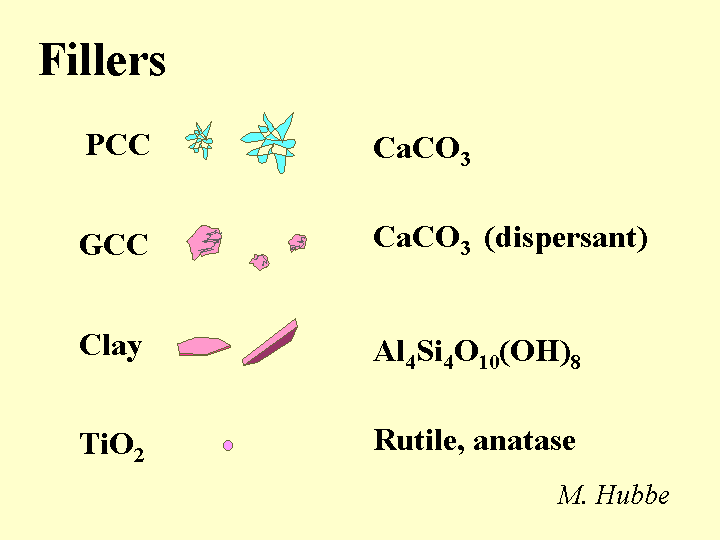
FLUORESCENT WHITENING AGENTS (FWAs)
Composition: Members of this class of diaminostilbene sulfonate derivatives are highly conjugated molecules having planar structure and anionic charge. All of these adjectives also apply to direct dyes. What makes this particular class of direct dyes different is that they absorb ultraviolet light and re-emit light in the blue region of the visible spectrum. Another traditional term for fluorescent whitening agents (FWAs) is “optical brightening agents” (OBAs). Three general types of FWAs are widely available. The type most often used by papermakers has four sulfonate groups (tetrasulfonated). It has intermediate solubility in water and it is readily retained on fibers, especially if alum or another cationic material is present. Hexasulfonated FWAs don’t retain as well when added at the wet end, but they may give higher optical efficiency when used at the size press due to less association between the molecules in the dried starch film. Disubstituted FWAs are sometimes used for specialty purposes, for instance when water bleed-fastness is critical.
Function: Increasing the white appearance of papers by absorbing invisible ultraviolet light and re-emitting it in the blue region of the visible spectrum. This strategy can compensate for a yellow tint of many types of pulps that have been bleached to moderate levels.
Strategies for Use: Some of the critical factors in FWA use include (a) retention, (b) quenching, (c) competition with other UV-absorbers, and (d) metamerism. The strategies for adding and retaining FWAs to the wet end of a paper machine are very similar to those used with direct dyes. For instance, FWA retention can be increased by sequential addition of alum to the pulp stream, either before or after the whitener. But there is a key difference; highly charged cationic polyelectrolytes easily can destroy the fluorescent character of the molecule. The effect is called “quenching.” (The effect is related, but much more problematic, than the tendency of the highly cationic polymers to modify the hue of certain direct dyes.) Both lignin and titanium dioxide are potent absorbers of ultraviolet light. For this reason, internal addition of FWAs to high-yield furnish or to furnish that contains TiO2 is likely to be ineffective. This is one of the reasons why it is common for papermakers to add all or most of the FWA at the size press. The idea is that light first encounters material nearer to the surface of the paper, and this is where much of the size-press formulation ends up. Metamerism refers to the phenomenon that two objects may appear to have identical color when viewed under a certain type of illumination, but the same objects might not match under a different lighting. This is a very common occurrence in the case of samples that contain different levels of activity of fluorescent whitening agents.
Cautions: The material is not known to be particularly hazardous. It may sometimes become a target of concern for health because of the fact that its presence is less obvious than is true for “normal” dyes that absorb visible light.
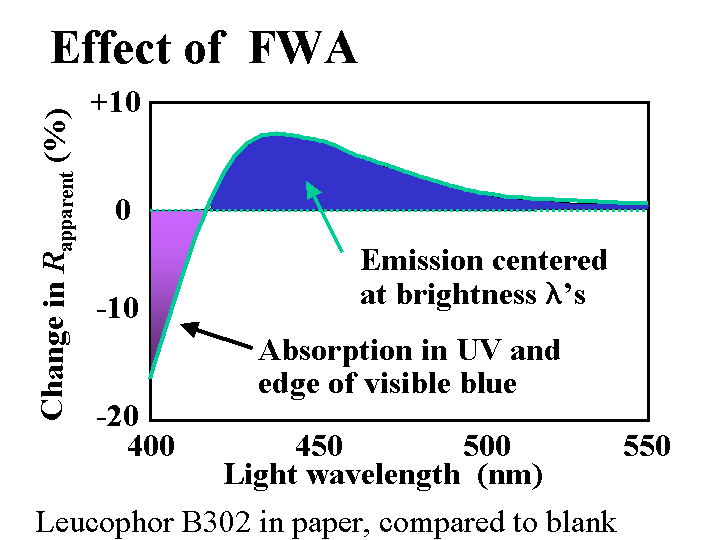
GROUND CALCIUM CARBONATE (GCC)
Composition: When papermakers use the term “GCC” they are usually referring to ground limestone, a calcium carbonate material having the calcite crystal structure. It is possible that they might be referring to a type of calcium carbonate called chalk, but the word “chalk” itself is also in common use. Limestone formations are formed by natural precipitation, a process that occurs slowly. This is part of the explanation why the material in limestone products is completely converted to the CaCO3 form. By contrast, depending on the conditions of its production, precipitated calcium carbonate may contain some residual calcium hydroxide. Because of the hardness of limestone, the grinding process is energy-intensive. Anionic dispersants, including phosphates and acrylates, are used as grinding aids and to make the resulting slurry more stable in a colloidal sense. Papermakers commonly use ground limestone products that are “fine-ground” or “ultrafine-ground” with equivalent particle sizes from 60% to 90% smaller than 2 mm based on sedimentation rates.
Function: For filling of paper for cost reduction, brightness, buffering in the alkaline pH range, and some opacity
Strategies for Use: Like any other calcium carbonate filler, GCC is incompatible with acidic conditions. Alum or sulfuric acid, etc., must never be added to a stream of GCC slurry. GCC can be obtained from high-quality limestone sources, yielding a product of very high brightness. Compared to the rosette form of PCC, GCC tends to produce a denser paper sheet with higher tensile strength at a given filler level. The strength advantage of GCC can be further improved if one uses a product whose particle size distribution has been narrowed by separation operations after grinding. PCC is often preferred in grades that require maximization of caliper at a given smoothness value. In other cases there may be better runnability and economy by replacing some or all of the rosette product with either GCC or rhombohedral (blocky shaped) PCC particles. The point of addition for GCC slurry can be more related to process control than chemical issues. Effective control requires that at least a significant fraction is added to the thin-stock, just several seconds ahead of where the furnish begins to become paper. A retention aid system should be used to maintain acceptable Z-directional uniformity, basis-weight uniformity over time, and efficient performance of ASA or AKD sizing agents.
Cautions: Ground limestone is generally non-hazardous. Unstirred tanks and pipes containing stagnant GCC slurry may become the site of dense sedimentation, that is very difficult to resuspend.
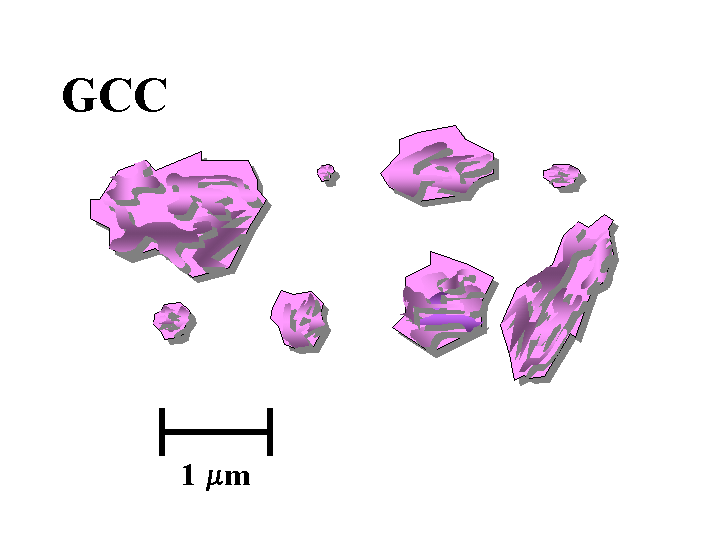
HARDWOOD
Composition: The major component of wood from a deciduous (hardwood) tree consists of libriform fibers. These are about 1 mm in length and about 20 micrometers in width. Wood from deciduous trees is a complicated mixture of these fibers, multicellular vessels (for transport of water from the roots), and other specialized cells. The kraft pulping method chemically dissolves the lignin, a natural phenolic resin that glues fibers together in wood. Kraft pulping yields fibers that tends to have superior bonding ability after they have been made flexible by the compression and shearing action of a refiner. The down side is that kraft pulping may remove more that half of the original mass of wood. By contrast semi-chemical hardwood pulps are cooked under conditions that solubilize only a fraction of the lignin. Some mechanical refining is needed after the semi-chemical cook to liberate fibers from the softened chips. Two such processes are the carbonate cook and neutral-sulfite semi-chemical (NSSC). Because lignin is stiff, these pulps behave differently. Also, the high content of lignin and extractable materials such as fatty acids contributes to a higher density of anionic surface charge of semichemical fibers, compared to most kraft pulps.
Function: Hardwood fibers are especially suited for producing smooth papers for printing and writing. They also can achieve good stiffness and bulking ability, but this depends on the tree species and refining conditions. Hardwood pulps also tend to produce paper products with more uniform formation, compared to softwood fibers.
Strategies for Use: Papermakers are well aware that different blends of fiber types make sense for different grades of paper. If one wishes to manufacture paper bags it would be foolish to consider using hardwood pulps; they simply would not have enough resistance to tearing. On the other hand, if one wishes to make fine writing papers, it would be foolish to consider 100% of the relatively large southern softwood fibers. The sheet would be too rough and too porous. But wait – if one were to use no softwood fiber at all in making that same writing grade, then it is quite likely that the web will be too weak to run efficiently on your paper machine. One of the most interesting cases to consider is that of the common corrugated box. The flat layers (the “linerboard”) are made from unbleached softwood kraft pulp to maximize resistance to edge-wide compression failure. However the interior corrugated or fluted part (the “medium”) is usually made out of high-yield hardwood semi-chemical pulp. The relative high content of lignin in such fibers means that they will be stiff enough to play their essential role in keeping the two liners spaced at an optimum distance for strength. When two fiber types are to be blended, it is often the case that the best overall mix of properties can be achieved by separate refining of each type. For example, most softwood pulp can benefit from much greater refining energy input, compared to hardwood. Over-refining tends to reduce fiber strength greatly and produce fines that impede dewatering from the paper.
Cautions: Hardwood fibers and slurries of these fibers are usually non-hazardous if one follows usual safe practices.
Wood fiber types, showing aspect ratios and shapes of hardwood and softwood
KAOLIN
Composition: This discussion will be limited to kaolin, the most common form of clay added to paper as a filler. (Bentonite, a generic term for montmorillonite clay, is a microparticle, and it is described elsewhere in this mini-encyclopedia.) Kaolin is a hydrated silica-aluminate. Deposits of kaolin particles have been built up by natural geologic processes. In one of these processes the kaolinite first becomes released by glaciation. Rivers carry silt in the glacial runoff and deposit it in shallow seas. Larger particles settle first, then the finer ones, usually at a different location. By choosing their locations, clay producers have access to a range of particle sizes and brightness of kaolin particles. High quality deposits of kaolin, having moderately high brightness and uniform particle size are found all over the world, but especially in China, Georgia, Brazil, and England. Anionic dispersants such as phosphates and acrylates are usually added to clay as it is collected and processed. The particles are typically irregular hexagons with a layered underlying structure. The size is highly variable; filler clays usually are selected with an average (SEM) particle size of about 1 to 3 micrometers. When the kaolin is first released it is likely to contain dark and abrasive impurities. Processing steps can include sieving, centrifugal cleaning, magnetic removal of dark contaminants, and bleaching. After these processes the particles of kaolin is still likely to resemble “books,” comprised of layers. This is the most common type of clay used for paper filling, especially when it is important to maintain strength at a given filler level. Extended agitation of clay slurries in the presence of hard ceramic balls (of visible size, much larger than the kaolin particles) causes the kaolin to delaminate into thinner platelets. This is delaminated clay.
Function: Besides reducing the cost of paper, clays are especially useful for creation of gloss (in highly filled, supercalendered papers), for increasing resistance to air flow (in the case of delaminated clays), and for imparting a moderate decrease in the friction coefficient of paper (especially when added to the size-press solution).
Strategies for Use: The main concerns are (a) how high a filler level is best, and (b) how to achieve an adequate retention. A uniform dispersion of clay can be obtained with conventional dispersing equipment, e.g. a Cowles mill. Also it is possible to get bulk delivery of clay slurries with solids levels in the neighborhood of 70%. The price that one pays for the convenience of using pre-slurried clay is that it contains anionic dispersants. These can make retention more difficult. Coating grades of clay are especially difficult to handle at high levels in the wet end due to the combination of dispersants, higher surface area, and smaller particles that are harder to filter from the water as the paper is being formed. The content of clay in paper usually is limited by decreasing strength properties and decreasing caliper at a given smoothness and basis weight. Clay tends to produce dense paper, especially when it is calendered. Unlike calcium carbonate fillers, clay products can be used at any pH. Clay will form agglomerates if prematurely mixed with such additives as cationic starch, alum, retention aids, and the like. Intentional agglomeration of filler is a potential way to increase the strength of paper at a given filler content; however this technology has not become widely used. Most retention aid systems are effective with clay. Ashing of the paper at 900 oC drives off waters of hydration. If a paper sheet is assumed to contain only hydrous kaolin (not calcined clay), then the percent ash needs to be divided by 0.86 to estimate the percent of clay originally present in the paper.
Cautions: Normal precautions regarding dust need to be observed when clay is received as a dry powder.
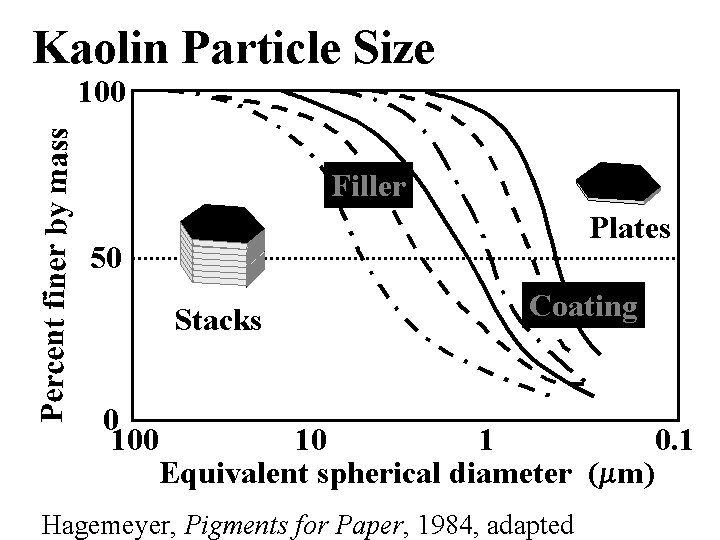
KRAFT PULP
Composition: Kraft pulp is what you get after you place a chip of wood in a pressurized vessel in the presence of hot caustic soda and sodium sulfide. The cooking process attacks and eventually dissolves the phenolic material called lignin that glues the fibers to each other in the wood. The word “kraft” means “strong” in the language of its origin. German manufactures of pulp discovered that addition of the sulfur to a “soda cook” improved the selectivity of the process – dissolving the lignin with less damage to the cellulose. The fibers initially liberated by kraft pulping usually are brown, similar to a typical grocery bag (paper type) or corrugated box. Bleaching of kraft pulps requires a series of aggressive chemical treatments with oxidizing agents and caustic soda. In either case, kraft pulps tend to be more flexible than fibers produced by purely mechanical refining of pulp. The superior bonding potential of kraft pulps becomes evident when they are refined sufficiently to allow collapse of the lumen, delamination of the cell wall, and partial fibrillation of the surfaces. Kraft fibers tend to be more porous, compared to mechanical fibers. Slit-like pores having widths in the range of 5 to 20 nm appear to occupy the space formerly occupied by lignin. Each time that paper is dried, many of these pores appear to close irreversibly. Under ordinary condition of repulping, the fibers do not regain their original ability to swell with water.
Function: Main structural element in many paper grades, including corrugated boxes, office papers, liquid cartons, high-grade folding boxboard, labels, bags, cups, and many more. Grades such as magazine paper, even if they contain mostly mechanical fibers, are likely to contain some softwood kraft pulp to achieve needed levels of fold resistance and tear strength.
Strategies for Use: One of the keys to successful use of kraft pulps is optimization and uniformity of refining conditions. Refining usually is needed in order to meet bonding strength objectives. However, too much refining causes excessive damage to individual fibers, yielding paper that may be too thin or too easy to tear. In such cases dry-strength additives such as cationic starch may be used in order to meet bonding strength requirements with less loss of bulk and tear strength. Uniform refining can be achieved by a combination of approaches. These include control of the consistencies and flows to a refiner, control of the amount of energy expended per dry-mass of pulp, and on-line freeness control (usually not available). Higher pH conditions (in the range up to about 8) favor more rapid refining with less damage to the fibers, especially in the case of high-yield kraft pulps. Hardwood and softwood can be refined separately, then recombined, since the softwood usually can benefit from more severe or prolonged mechanical treatment. In general, a kraft pulp blend that is rich in softwood fibers (average length about 3 mm) is easy to run on a paper machine and the paper is tough. A kraft pulp blend rich in hardwood fiber is less forgiving of non-ideal tensions in open draws on the paper machine and the product is more easily torn.
Cautions: Kraft fibers and slurries of these fibers are usually non-hazardous once they are delivered to the paper mill. Various toxic materials such as sodium sulfide and chlorine dioxide are used in their preparation.
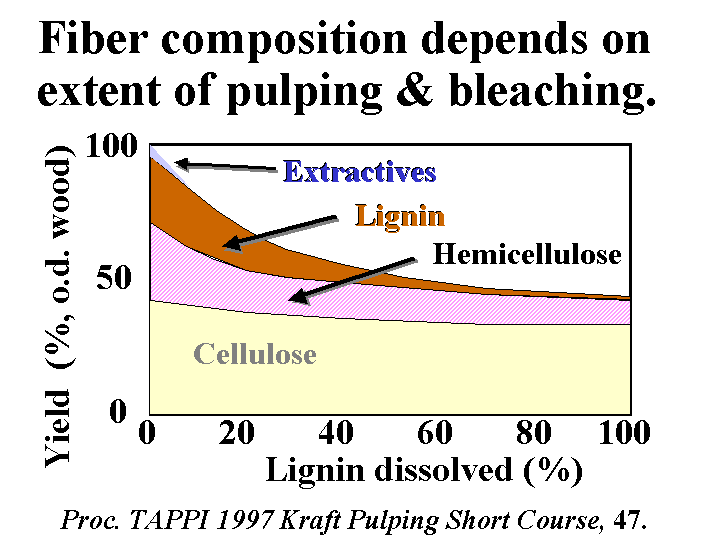
MIXED OFFICE WASTE (MOW)
Composition: “Engine blocks, dead cats, dead skunks, you name it…” But the main contents of mixed office waste are xerographic papers and offset papers. Inks are the first types of contaminants to consider. Other common contaminants of “MOW” include labels, carbonless forms, unbleached kraft, paper made from mechanical pulp, and colored papers. The contaminants present in the second list make a difficult situation worse, and many recyclers take great pains to sort their incoming supply. The usual approach is to disperse the bales of MOW in a hydropulper, remove very large and heavy contaminants with the centrifugal action of a large hydrocyclone, screen the pulp, wash it, and possibly use flotation to remove fine particles. Since it is difficult to remove all of the ink, mixed office waste is often bleached with hydrogen peroxide. Xerographic toner, especially when it is applied by computer-generated “laser printers,” can be difficult to detach from fibers. Various surface-active materials are used to promote release and either disperse the particles or cause them to agglomerate. Even this brief review of the steps in de-inking of mixed office waste should make it evident that the product of de-inking might be quite different from the bleached kraft pulp that was used to make most of the original products that were the sources of the fiber. Some materials that are likely to be present in de-inked MOW include residual toners and ink, stickies (from labels and binding of magazines), surfactants, hydrogen peroxide bleach residual (if de-inked on-site), silicates (a stabilizer for peroxide), and some mineral filler that makes it through de-inking.
Function: The goal of most papermakers is to use recycled MOW pulp in place of some or all of the virgin bleached kraft fibers in various grades of paper.
Strategies for Use: Some of those who have done the most careful life-cycle analyses of paper recycling have suggested that office waste should be burnt in highly efficient incinerators, taking the place of fossil fuels that otherwise might be expended for the generation of energy. However, others point to the fact that the fibers contained within mixed office waste represent the culmination of a capital-intensive pulping process, and it is wasteful to just throw such valuable fibers away. Because of the many steps needed to “clean up” mixed office waste, the process can produce an amount of sludge that is about equal to the weight of paper product. Increased dosages of such additives as talc (to detackify stickies), biocides, and defoamers may be needed to keep the system running efficiently when the content of de-inked MOW is increased. Sizing agent dosages may have to be increased as well in order to overcome the effect of surfactants.
Cautions: Mixed office waste may contain decaying food products and other substances that carry germs.
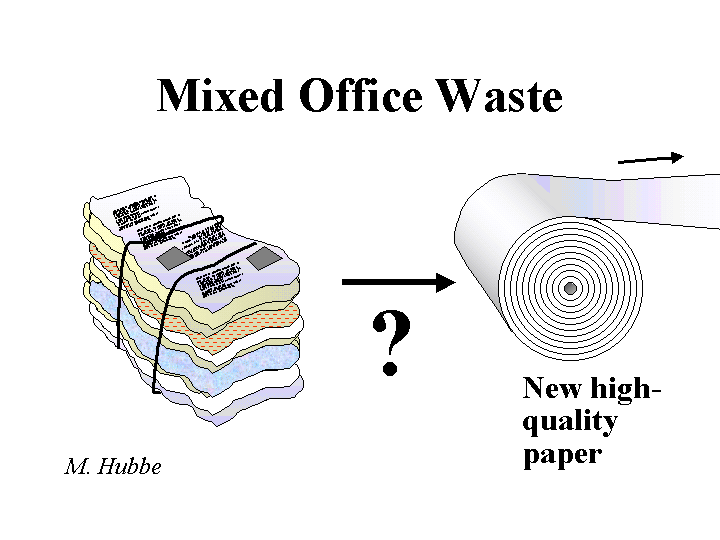
MONTMORILLONITE (bentonite)
Composition: Bentonite is a common name for the anionic smectite clay minerals used by papermakers as a retention and drainage additive or “microparticle.” Under the scanning electron microscope (SEM), bentonite particles can be almost indistinguishable from kaolin filler clay or coating clay. The main difference is the thickness. The sodium or potassium salts of bentonite exfoliate into extremely thin plates. In theory these plates can be as little as about 1 nm in thickness, yielding a huge surface area per unit mass. In practice, not all of the platelets will become separated from each other.
Functions: Retention and drainage (if added after or before a cationic retention aid); pitch control (if used in combination with highly charged cationic polymers).
Strategies for Use: First let us consider the role of bentonite as a retention and drainage promoter. Following the patented technology of Ciba Specialty Chemicals, bentonite added downstream of a cationic polyacrylamide retention aid causes a marked improvement in dewatering. Best results are achieved when sufficient very-high-mass cationic polymer (e.g. cationic acrylamide) has been added or is about to be added downstream so that the furnish momentarily has a net cationic charge. If the furnish has a high level of anionic dissolved and colloidal materials, then it makes sense to first treat the stock with a highly charged cationic material to neutralize most of this excess charge. The high aspect ratio of bentonite means that it has significant ability to bridge between different particles or fibers covered by the cationic polymer. That explains why bentonite tends to be very effective for retention, as well as for improving drainage. Patented technology from Vinings, Inc. shows that bentonite can be used in combination with highly cationic polymers for adsorption and detackification of pitch.
Cautions: When handling dry materials it is important to take proper precautions against airborne dust.
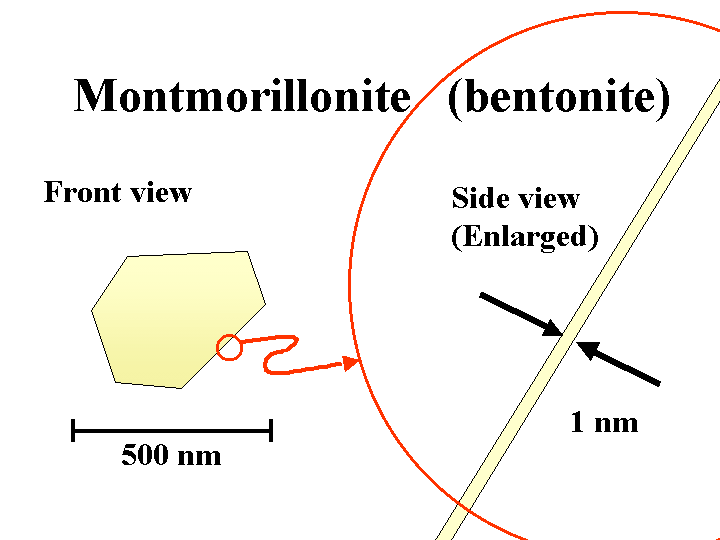
OLD CORRUGATED CONTAINER PULP (OCC)
Composition: Four main components of “old corrugated container” (OCC) pulps are unbleached softwood kraft pulp (mainly from the linerboard), semi-chemical hardwood pulp (from the fluted medium), starch (as an adhesive), and water (often 8% or more).
Function: OCC pulp is often used for the production of linerboard. It is worth noting that the high-yield hardwood from the corrugated layers of boxes is not ideal for making linerboard, since the fibers are not as long and they don’t bond as well as softwood kraft.
Strategies for Use: Papermakers employ such steps as hydropulping, high-density hydrocyclone cleaning, thermal dispersion, flotation, and washing or thickening to remove contaminants. The pulp is refined and cationic starch is added as needed to achieve the target strength properties. The expected slower drainage of recycled furnish, especially after the repeated refining treatments, may require changes to the forming section of the machine, e.g. the addition of a top-wire section to a Fourdrinier machine. Alternatively, inadequate dewatering can be addressed by use of drainage aids. Wax-containing corrugated boxes (for fruits, chickens, etc.) should be separated from the incoming supply to avoid contamination.
Cautions: Recycled unbleached kraft fibers and slurries of these fibers may contain hazardous contaminants.
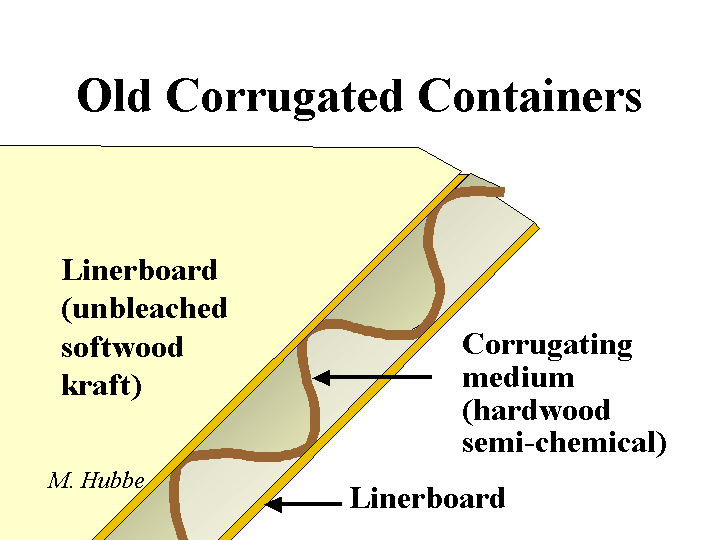
The net fiber composition of typical OCC pulp collected in the U.S. can be traced to its origins in two different kinds of plies.
OLD NEWS PAPER PULP (ONP)
Composition: “ONP” pulp consists mainly of old newspapers. But also it includes coated inserts and a moderate level of other types of paper and contaminants. The newsprint portion is mostly mechanical fiber, containing most of the dry content of wood. In the original production of the pulp the wood was converted either by grinding (the traditional approach) or by thermomechanical refining of chips (TMP). These mechanical pulping processes produce a wide distribution of fibers and fragments of fibers. A minority of the newsprint paper may have been upgraded by hydrogen peroxide bleaching, hydrosulfite bleaching, or by the addition of some clay to improve the brightness and opacity. Because ONP may have been exposed to sunlight, some of it may be considerably more yellow than fresh newsprint pulp. The most common form of ink applied to newspapers consists mainly of carbon black particles dispersed in a heavy oil. Only in the case of premium newspapers does the ink contain soybean oil or additives to promote drying or setting. Rather, the oil mainly absorbs into the fibers. The surface charge of ONP, after it has been dispersed in water, tends to be highly negative due to the presence of wood extractives, dispersants from the coatings on various glossy inserts, and byproducts of peroxide bleaching. Dirt and stickies are partly removed, in most cases, by screening and cleaning.
Function: The main component of recycled newspapers. Also used in other recycled paper and paperboard products where low cost is a main concern.
Strategies for Use: The papermaker may need to modify the process in order to run ONP in place of virgin mechanical pulp. The extent of these modifications depends greatly on how well the pulp has been washed, screening, and cleaned after repulping. Talc and other detackifying agents may be increased in order to handle the variable load and tackiness of the mixture of latex, polyvinylacetate (from labels), and wood pitch. It is likely that the furnish will have different drainage characteristics; again this depends on the extent to which fine materials may have been washed from the furnish during the removal of contaminants. De-inked newsprint pulp may contain surfactants, including fatty acids that are not unlike those originally in the wood. Papermakers have a range of options, including treatment with highly cationic polymers (for charge neutralization and to improve drainage), cationic starch (for strength), and retention aids (to keep the system clean). Also there is likely to be an increased requirement for biocides.
Cautions: A program of safe operating procedures in the mill will include the safe handling of ONP.
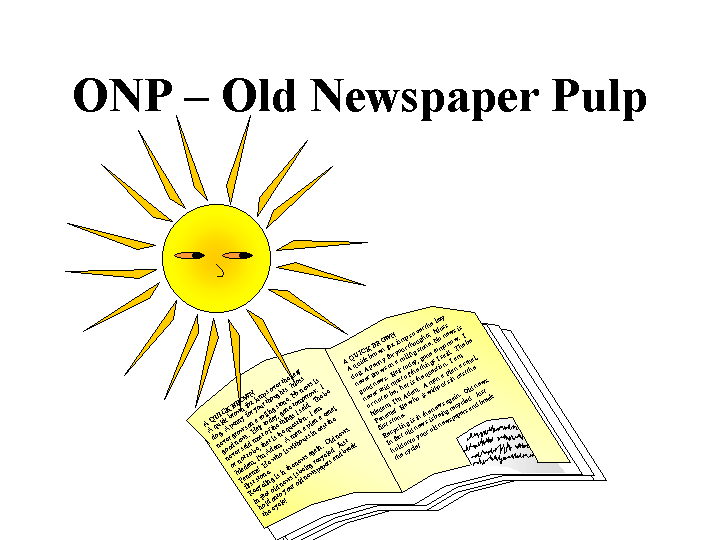
Cartoon illustrating the rapid yellowing of mechanical fiber exposed to sunlight. Clipping of a coupon has revealed a non-yellowed sheet below the top sheet.
OPTICAL BRIGHTENERS (fluorescence whitening agents)
Composition: Members of this class of diaminostilbene sulfonate derivatives are highly conjugated molecules having planar structure and anionic charge. All of these adjectives also apply to direct dyes. What makes this particular class of direct dyes different is that they absorb ultraviolet light and re-emit light in the blue region of the visible spectrum. Another traditional term for fluorescent whitening agents (FWAs) is “optical brightening agents” (OBAs). Three general types of FWAs are widely available. The type most often used by papermakers has four sulfonate groups (tetrasulfonated). It has intermediate solubility in water and it is readily retained on fibers, especially if alum or another cationic material is present. Hexasulfonated FWAs don’t retain as well when added at the wet end, but they may give higher optical efficiency when used at the size press due to less association between the molecules in the dried starch film. Disubstituted FWAs are sometimes used for specialty purposes, for instance when water bleed-fastness is critical.
Function: Increasing the white appearance of papers by absorbing invisible ultraviolet light and re-emitting it in the blue region of the visible spectrum. This strategy can compensate for a yellow tint of many types of pulps that have been bleached to moderate levels.
Strategies for Use: Some of the critical factors in FWA use include (a) retention, (b) quenching, (c) competition with other UV-absorbers, and (d) metamerism. The strategies for adding and retaining FWAs to the wet end of a paper machine are very similar to those used with direct dyes. For instance, FWA retention can be increased by sequential addition of alum to the pulp stream, either before or after the whitener. But there is a key difference; highly charged cationic polyelectrolytes easily can destroy the fluorescent character of the molecule. The effect is called “quenching.” (The effect is related, but much more problematic, than the tendency of the highly cationic polymers to modify the hue of certain direct dyes.) Both lignin and titanium dioxide are potent absorbers of ultraviolet light. For this reason, internal addition of FWAs to high-yield furnish or to furnish that contains TiO2 is likely to be ineffective. This is one of the reasons why it is common for papermakers to add all or most of the FWA at the size press. The idea is that light first encounters material nearer to the surface of the paper, and this is where much of the size-press formulation ends up. Metamerism refers to the phenomenon that two objects may appear to have identical color when viewed under a certain type of illumination, but the same objects might not match under a different lighting. This is a very common occurrence in the case of samples that contain different levels of activity of fluorescent whitening agents.
Cautions: The material is not known to be particularly hazardous. It may sometimes become a target of concern for health because of the fact that its presence is less obvious than is true for “normal” dyes that absorb visible light.
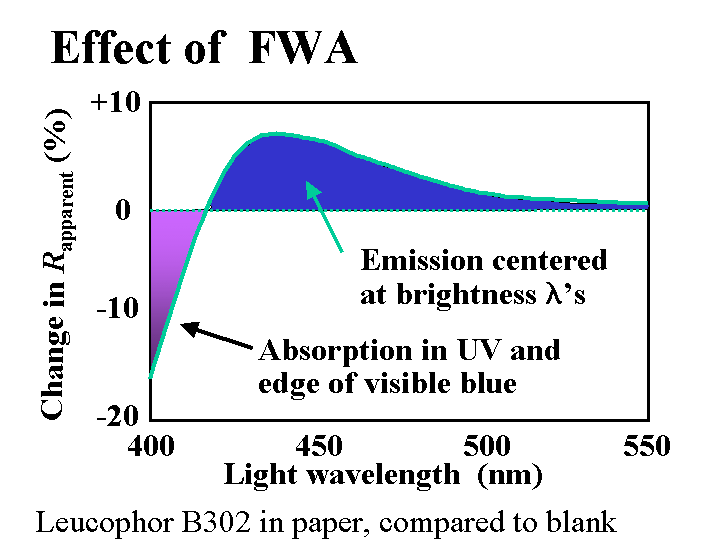
PITCH (wood resins)
Composition: When papermakers use the word “pitch” they are talking about tacky materials that usually contain resins from the wood. Other materials are likely to be present as well. Wood chips from different types of trees are likely to contain from about 1 to 5% of wood pitch. Usually a significant part of this pitch consists of fatty acids, and, in the case of softwood chips, resin acids. Either of these acids is easily converted to semi-soluble soap form as the pH is raised to the neutral range. A major portion of the pitch is likely to consist of non-ionic triglyceride esters of fatty acids; treatment with strong alkali, as in the case of kraft pulping and bleaching, is expected to hydrolyze the ester bonds, forming acid salts and glycerin. Wood pitch also contains non-saponifyable, oily substances such as beta-sitosterol. Materials from other sources tend to associate with pitch in the same agglomerates, deposits, and spots in the paper product. Susbstances that tend to associate with wood pitch include latex (from repulping of coated broke), components of defoamers (especially ethylene-bis-stearamid), and talc (often used as a pitch-control agent).
Function: Most of the resin contained within a paper sheet causes no difficulty either to the producer or to the user of the paper. Deposition of even a fraction of a percent of the pitch onto papermaking equipment can force an operation to close down for frequent, intensive cleaning (“boilouts”) or take expensive measures to control the pitch.
Strategies for Use: Pitch problems can be very complex due to the many different hydrophobic materials that can associate with each other in a paper machine furnish. For this reason, it is highly recommended to do a chemical analysis. This may make it possible to determine the most likely root causes of the problem. One of the most general strategies for avoiding pitch problems is to keep the tacky materials bound to the fibers. This is one of the ideas behind a common practice of adding alum during or immediately after mechanical pulping. The alum complexes with any soaps of resin acids of fatty acids, and the presence of these complexes can serve to bind the multi-component droplets of pitch to the fiber surfaces. Addition of highly cationic polymers such as polyethyleneimine (PEI) to the process can have a similar effect. The relative turbidity of filtrate obtained from different points in the process can be used as an indication of the probable effectiveness of such treatments with cationic materials. It is very common also to add talc or another detackifying agent to coat tacky particles and minimize their tendency to form large agglomerates, deposits, or spots in the product. An effective retention aid system can help limit the filling of press felts with pitch. Spraying the forming fabric with a very dilute solution of highly cationic polymer can create a hydrophilic layer that resists deposition of oleophilic materials. Wet-press felts can rinsed either continuously of periodically with solutions that may include nonionic surfactants, alkali, and solvents.
Cautions: Safe procedures must be followed when collecting samples of pitch deposits. Equipment must be locked out and the air quality checked by qualified people before entering enclosed areas such as headboxes.
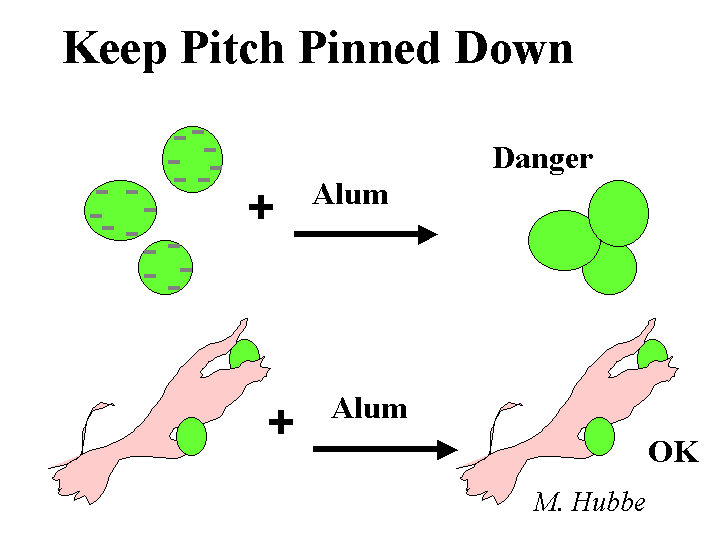
POLYACRYLAMIDE
Composition: Though the word “polyacrylamide” is more accurately applied in the case of the homopolymer, papermakers tend to use the term when talking about the most popular varieties of cationic and anionic retention aids. These are actually copolymers of acrylamide (main component) and charged co-monomers (usually minor component). Acrylamide products used as retention aids typically have molecular masses in the range 5 to 20 million grams per mole. Acrylamide products used as dry-strength agents typically have molecular masses in the hundreds of thousands. The cationic copolymers usually have no more than 5% of cationic groups due to FDA limits for paper products that may contact food. The anionic copolymers also can be prepared by an alternative route, partial hydrolysis of the amide groups after synthesis of the polyacrylamide homopolymer. Retention aids are commonly produced and delivered to the mill site in the form of a water-in-oil emulsion. The emulsion needs to be inverted with good agitation and a dilution ratio of approximately 100 to 1. Dry-strength agents often are delivered as aqueous solutions that can be diluted with non-specialized equipment.
Function: Retention aids, dry-strength agents.
Strategies for Use: In their role as retention aids, acrylamide copolymers are added very late to the process, typically either right before or after the pressure screens. The pressure screens always degrade the molecular mass, hurting efficiency of retaining fine particles during paper formation, but the paper is more uniform. In their role as dry-strength agents the most critical aspect of acrylamide copolymer use is to balance the colloidal charge. Typically an oppositely charged material has to be added to the furnish either before or after the strength agent. For control of pitch it is often recommended to treat the offending stream of thick-stock with just enough polymer so that filtrate from that stream has a low turbidity. Micro-polymers (see also microparticles) usually are added last to the process, after a very-high-mass retention aid of opposite charge. One of the most critical factors to the success of a microparticle program is that the furnish should have the same net colloidal charge as the retention aid at that point (usually by adding a charge scavenger).
Cautions: These materials can be very slippery when spilled. The emulsions of very-high-mass retention aids are particularly hazardous in this regard. Spills should be first wiped up with dry absorbent (think “cat litter”).
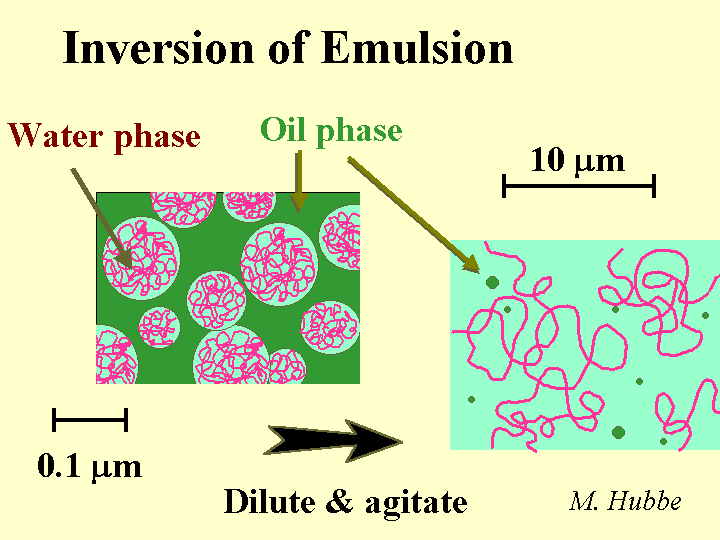
POLYALUMINUM CHLORIDE (PAC)
Composition: The term “poly-aluminum chloride” or “PAC” refers to a class of soluble aluminum products in which aluminum chloride has been partly reacted with base. The relative amount of OH-, compared to the amount of Al, determines the basicity of a particular PAC product. The chemistry of PAC is often expressed in the form Aln(OH)mCl(3n-m). Basicity can be defined by the term m/(3n) in that equation. Because PAC is more expensive to produce than papermakers alum (aluminum sulfate) it is important to note the most important differences between these two products. Solutions of PAC are not as acidic as alum; consequently they do not tend to decrease the pH of the furnish nearly as much as an equivalent amount of alum. Another difference is that PAC is formulated so that it already contains some of the highly cationic oligomers of aluminum – materials that are especially effective for the modification of colloidal charges in a papermaking furnish. A particularly stable and important ionic species in PAC and related soluble aluminum chemicals has the formula Al12(OH)24AlO4(H2O)127+.
Function: To boost drainage rates especially in neutral and alkaline papermaking, to help with certain retention aid strategies, and for neutral sizing with certain rosin-based emulsion products. PAC products of low (0 to 17%) and medium (17 to 50%) are commonly used in papermaking applications, whereas PAC products of higher basicity are useful for water and wastewater treatment.
Strategies for Use: Because PAC is less acidic than alum there is less danger of shocking the system with a pH change at the point of addition. That means that papermakers have a certain degree of flexibility regarding the point of addition. An example worth considering is the case of virgin unbleached linerboard, when it is made under acidic papermaking conditions. The pulp often contains a significant level of barium compounds. Addition of alum depresses the pH, converting Ba(OH)2 to soluble divalent barium. The barium ions build up in the system until the solution is supersaturated with respect to barium sulfate. Insoluble, hard, barium sulfate scale then precipitates from solution onto solid surfaces exposed to high levels of shear. Substitution of PAC for alum in such cases can reduce the problem considerably by decreasing the amount of pH drop and avoiding an additional contribution of sulfate ions. One has always to be careful of the dosage of PAC, since an overdose is expected to convert the net colloidal charge of the system to a positive sign. In the presence of calcium carbonate filler, the use of PAC in place of alum is likely to reduce the amount of dissolution of the calcium carbonates.
Cautions: Some papermakers express concern that the chloride content of the product may accelerate corrosion of stainless steel, especially in cases where such corrosion occurs beneath deposits.
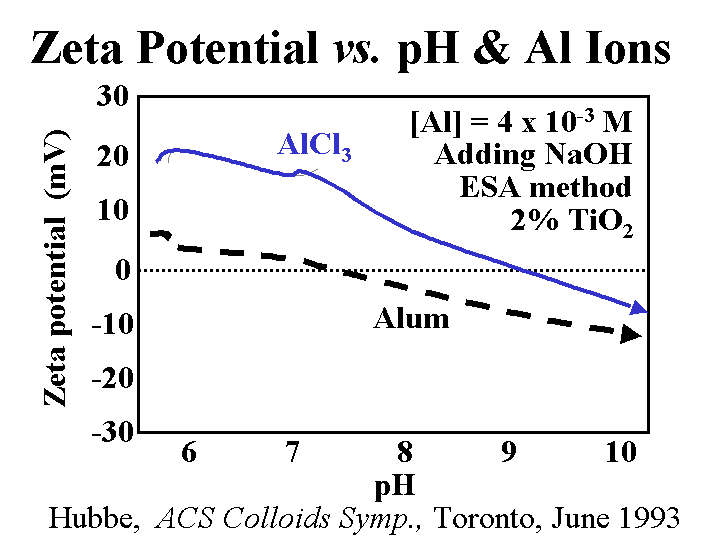
Aluminum chloride was found to have approximately the same effect on zeta potentials as PAC.
POLYALUMINUM SILICASULATE (PASS)
Composition: A liquid concentrate called “PASS” can be formed by treating an alum solution with a silicate compound. If such an addition were to be continued too far, then it would result in formation of insoluble aluminum silicate. But it has been discovered that partial neutralization of alum’s acidity with silicate produces a meta-stable solution with many attributes similar to PAC. For instance, addition to a paper machine system has less impact on pH, compared to addition of an equivalent amount of alum. PASS is unique, however, in its hair-trigger readiness to flocculate and come out of solution. Simple dilution with water, depending on the pH, can result in formation of the characteristic “haze,” indicating the presence of colloidal particles. Such particles quickly lose most of their “activity,” – something related to their colloidal charge and surface area. Though most soluble aluminum products are noted for their ability to neutralize anionic colloidal charges, PASS usually has too high a degree of neutralization by the silicates to be cost-effective for that purpose alone.
Function: Water clarification, developmental applications in retention and drainage.
Strategies for Use: Because PASS is relatively new on the scene and rather different in character from other cationic inorganic products used by the industry, the best strategies for use are not yet well known. Logic would suggest that PASS is an excellent candidate to consider for applications where one has had difficulties achieving sufficiently rapid coagulation of a colloidal mixture. There is a good example of such an application in the field of water treatment; PASS is effective for water clarification at low temperatures. As noted above, it is not recommended to pre-dilute the product before it is added to a process stream; such dilution is likely to make the additive less effective as a flocculant.
Cautions: The product has limited shelf-stability. Manufacturer’s recommendations need to be followed regarding the conditions and time of storage.
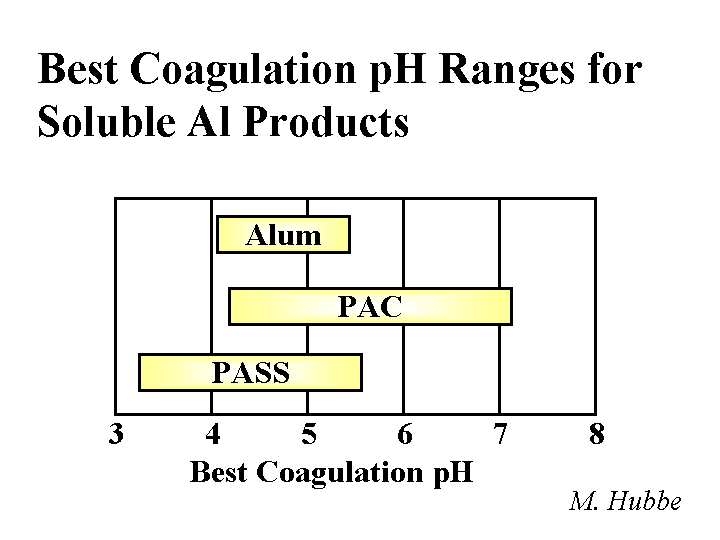
POLYAMIDOAMINE-EPICHLOROHYDRIN (PAAE)
Composition: This polyelectrolyte is formed by the reaction between adipic acid and diethylene triamine, and subsequent derivatization of the resulting copolymer with epichlorohydrin. Readers with a strong background in chemistry will recognize that the initial polymerization during the production of PAAE creates a chain very similar to that of Nylon. What’s different is that the chain contains secondary amine groups. Some of these react with the epichlorohydrin, and some of them remain in the product, helping to make it highly cationic and soluble under the conditions of use. The reaction with epichlorohydrin creates highly reactive aminochlorohydrin and azetidinium chloride (ring) groups along the chain. These groups appear to be capable of reacting with either the carboxyl groups at a cellulose surface, or amine groups on adjacent molecules of the additive during drying of paper. The groups added to the molecular backbone by reaction with epichlorohydrin are also highly cationic.
Function: Wet-strength agent especially well suited to neutral and alkaline papermaking; also sometimes used in formulation of cationic rosin size emulsions
Strategies for Use: The first consideration is shelf life. Storage for too long or too warm will cause PAAE to react with itself sufficiently that it looses its activity. Another thing to recognize from the beginning is that long exposure to hot papermaking furnish will reduce the performance of PAAE resin as a wet-strength agent. These factors would suggest that the best addition point would be close to the headbox of the paper machine. This would be true except for one other consideration; strength agents often are most effective when they are present on the fibers, rather than on fines. Since fines have surface areas at least three times that of fibers (on a mass basis), the fines are likely to adsorb a disproportionate amount of the additive. This effect is further amplified if one adds the resin in the thin stock – the part of the paper machine system where the relative concentration of fines in the furnish is at its highest. For that reason, strength resins often are added to the thick stock just before it is diluted at a fan pump. The performance of PAAE resin is strongly affected be the anionicity of the fiber surfaces. For this reason it can be easier to develop high levels of wet strength when using high-yield fibers. Another strategy to increase the anionic character of the fibers is to add the PAAE under alkaline papermaking conditions where the carboxyl groups on the fibers are mostly dissociated.
Cautions: Epichlorohydrin monomer may be hazardous, and suppliers of PAAE products have been able to reduce its presence to very low levels. Consult the MSDS.
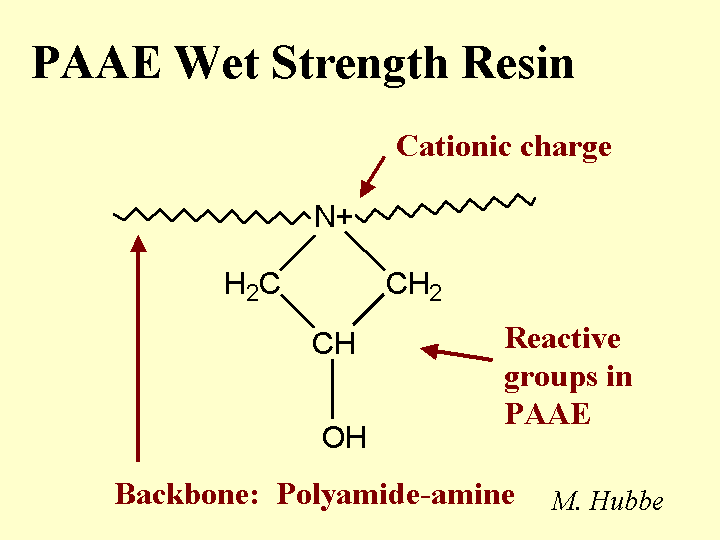
Structure of an azetidinium group, a source of cationic charge and reactivity on PAAE wet-strength resins
POLYAMINES (high-charge cationic polymers)
Composition: When papermakers speak of “polyamines,” they are most often referring to a series of copolymers of dimethylamine and epichlorohydrin. The repeating unit of the linear form of the copolymer is -CH2-CHOH-CH2-N+(CH3)2-. The presence of a quaternary ammonium group within the backbone of this molecule ensures that it maintains its very strong cationic charge throughout the pH range of most papermaking operations. Manufacturers can include other monomers to achieve branched versions of this molecule. Molecular masses are typically between tens of thousands and hundreds of thousands of grams per mole. The product is usually delivered in solution form, with a solids level between about 10% and 50%, depending on the molecular mass.
Function: Neutralization of excess anionic colloidal charge, especially in the case of neutral or alkaline papermaking; to establish anchoring points for an anionic polyacrylamide retention aid added later.
Strategies for Use: The use and optimization of highly charged additives to a paper machine is never simple. But there is one sense in which the behavior of polyamines is simpler than some other highly charged cationic additives such as alum or PEI. The simplifying attribute of the most commonly used polyamines is that their charge doesn’t depend on pH. The quaternary ammonium groups remain cationic throughout the pH values of acidic and alkaline papermaking. There are several things to consider when selecting an appropriate addition point. The first is the fact that these molecules need only a few minutes to partly absorb into the fine pores at the fiber surface. Such molecules then are unavailable with respect to retention and drainage mechanisms. In theory this can be avoided by adding the highly cationic material very late to the process. The other part of the story is that the charge balance of a papermaking furnish needs to be adjusted so that it is optimized and stable well ahead of the addition points for sizing agents, dyes, retention aids, and the like. Very late addition of polyamines sometimes can cause deposition of tacky materials such as dyes, defoamers, and pitch onto pressure screens and headbox surfaces. The best overall rule of thumb is to proceed in small steps with low dosages of polyamine either before or after the fan pump. There are two big exceptions to this rule. One is that polyamine might be added directly to one of the thick stock streams that has been identified as a major source of anionic charge. Another is that the polyamine might be used to precipitate dispersed pitch or stickies onto fibers in a thick-stock stream or chest where there is a high content of tacky materials.
Cautions: Refer to the MSDS and recommended procedures for each product.
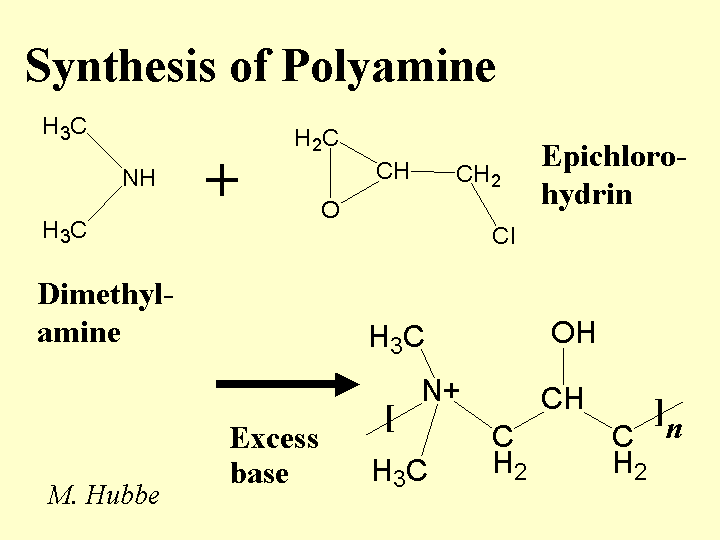
POLYDIALLYLDIMETHYLAMMONIUM CHLORIDE (DADMAC)
Composition: The name of this chemical says it all. It is a linear homopolymer formed from a monomer that has a quaternary ammonium and two unsaturated -CH=CH2 functionalities. The monomer itself is formed by reacting two equivalents of allyl chloride with dimethylamine. Free-radical polymerization of the “DADMAC” monomers yields a structure in which the quaternary ammonium groups are on rings that are included in the backbone of the polymer chain. This composition means that the poly-DADMAC macromolecules tend to be quite stiff, having a longer persistence length than, for instance, polyamines. For this reason, poly-DADMAC is expected to have a more extended conformation in solution. The molecular weight of DADMAC is typically in the range of hundreds of thousands of grams per mole, and even up to a million for some products. DADMAC is usually delivered as a liquid concentrate having a solids level in the range of 10 to 50%.
Function: Pitch control; charge neutralization; promoter for anionic retention aids
Strategies for Use: Highly charged additives, including poly-DADMAC products, should be well diluted before use. A concentration of about 1% is likely to be a safe starting point in applications where there is no previous experience. When poly-DADMAC is used for pitch control, the best strategy is to add it near to the main source of the problem. The idea is to anchor the tacky particles onto the fibers before they have a chance to collide with each other and grow. Though poly-DADMAC is very effective as a charge-control additive, trials may be needed to justify its higher cost (usually) compared to polyamines, and especially alum. Poly-DADMAC is sometimes added to furnish that later will be treated with an anionic polyacrylamide (aPAM) retention aid. The cationic polymer forms patch-like cationic sites on the fibers and fines that can serve as anchoring points for an anionic polymer.
Cautions: Read the MSDS and follow procedures recommended by the manufacturer
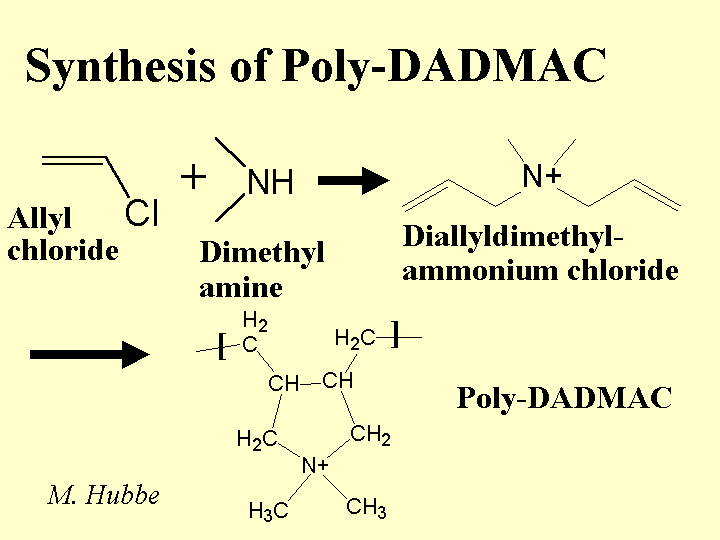
POLYETHYLENE IMINE (PEI)
Composition: The monomer is easy to describe. It consists of a three-membered ring. Two corners of the molecule consist of -CH2- linkages. The third corner is a secondary amine group, =NH. In the presence of a catalyst this monomer is converted into a highly branched polymer with about 25% primary amine groups, 50% secondary amine groups, and 25% tertiary amine groups. This product is sometimes called “pure polyethyleneimine” in order to differentiate it from certain copolymers of ethyleneimine and acrylamide. The latter mixture is copolymerized to produce so-called “modified PEI,” that has a molecular mass up to about 2 million grams per mole. Each of these types of products is delivered to the mill in the form of viscous solutions. Solids contents are the range of about 10 to 50%, depending on molecular mass.
Function: Pure PEI is very effective for neutralization of excess anionic colloidal charge, especially under acidic and neutral pH conditions. Modified PEI copolymers having relatively high molecular mass can be effective for drainage and pitch control.
Strategies for Use: The branched nature and exceptionally high charge of pure PEI make it an excellent choice for treatment of highly anionic furnish, furnish that has a high electrical conductivity, or thick-stock furnish. The branched structure is believed to provide some resistance to penetration of the molecule into very small slit-like pores in the fiber cell wall. The colloidal charge, filtrate turbidity, and drainage characteristics of the furnish should be monitored when selecting or optimizing PEI dosage. The modified PEI copolymer is usually added relatively late in the thin-stock cycle in order to achieve a significant boost in first-pass retention and the rate of drainage. Neither additive is expected to be cost-effective in furnish having pH values in excess of about 8, since the amine groups rapidly lose their charged character above that point. Likewise, strongly varying pH values are expected to cause variations in the performance of PEI products.
Cautions: Read the MSDS and follow procedures recommended by the manufacturer.
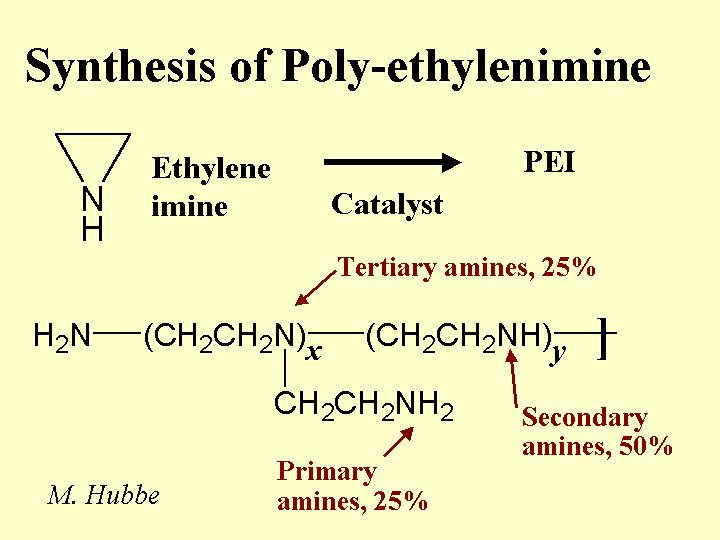
POLYETHYLENE OXIDE (PEO)
Composition: The ethylene oxide monomer is nothing more than an epoxide ring. Two corners of the molecule consist of -CH2- linkages. The third corner is an oxygen, -O-. In the presence of a catalyst the monomer forms a chain having the repeat unit -CH2-CH2-O-. The PEO products found to be most effective in various papermaking applications are those having very high molecular mass, much in excess of one million grams per mole. Factors that make PEO wet-end chemistry puzzling include (a) the fact that it can be used as a retention aid even though it is nonionic, (b) the fact that its performance is highly dependent on its shear history, and (c) the fact that it needs the presence of lignin or certain phenolic “cofactor” additives to achieve its best effect as a flocculant and retention aid. Observations of PEO behavior suggest that the molecular chains are initially tangled with each other and that this tangling is somehow essential for effective flocculation. PEO is usually received as dry granules. These need to be dispersed with care, using a dilution ratio of at least 100 and avoidance of excessive shear.
Function: Potent retention aid for high-yield pulps and for furnish to which a phenolic cofactor has been added; in theory it can be used as a formation aid for specialty applications where there is an insignificant amount of lignin or cofactor and where the drainage rate is not a concern.
Strategies for Use: Polyethylene oxide has been used as a retention aid since the 1970’s in various Canadian newsprint mills and other applications with high-yield pulps. In most of these cases the retention efficiency is maximized by first adding various proprietary phenolic compounds that appear to interact with the PEO and allow it to bridge between the adjacent solid materials in the furnish. As noted above, attention needs to be paid to the make-down process, using plenty of dilution and avoiding excessive or prolonged hydrodynamic shear. On the paper machine PEO needs to be trialed in small steps, since overdose can be expected to produce large fiber flocs that hurt the uniformity of the paper. A great potential advantage of PEO is that it is not affected by conditions of anionic charge or electrical conductivity. In other cases it has been reported that addition of highly cationic materials to the furnish ahead of PEO can improve the overall retention and drainage. In principle PEO ought to work as a retention aid in a highly closed white water system. The ratio of cofactor to PEO usually needs to be maintained within an optimum range. The possibility of using PEO as a “formation aid” is listed here only as a matter of completeness. It is known that very high mass polyelectrolytes having little affinity for fibers can be used to improve the uniformity of non-woven fabrics. If this approach were used with cellulosic fibers in the production of paper, it would be expected to reduce dewatering rates significantly, requiring a reduction of machine speed.
Cautions: Consult the MSDS and supplier recommendations. PEO is not expected to be particularly hazardous.
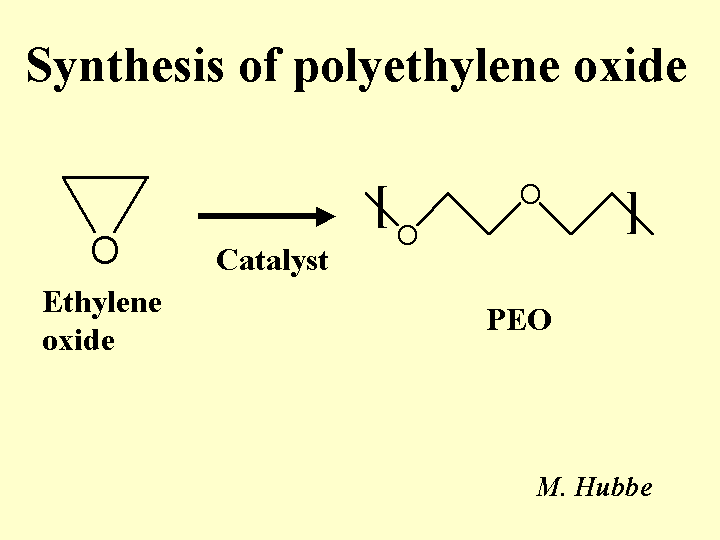
PRECIPITATED CALCIUM CARBONATE (PCC)
Composition: Most PCC added to the wet end of paper machines consists almost entirely of the calcite crystal form of CaCO3. The calcite crystal can have several different macroscopic shapes. These depend on the conditions of production. The most economical way to get PCC at a paper mill requires a satellite facility. The starting material may consist of limestone, but this is usually calcined (heated to drive off CO2) at the mine, producing burnt lime, CaO. The great advantage of CaO is that is has only 56 percent the mass of the original CaCO3, reducing the cost of shipping. Water is added to “slake” the lime at the satellite plant next to the paper mill. The resulting “milk of lime,” a suspension of Ca(OH)2, is then exposed to bubbles of CO2 gas. Often it is possible to collect a stream of CO2 gas from a kraft recovery operation or power generating operation at the mill site. Cool temperatures during addition of the CO2 tend to produce rhombohedral (blocky) PCC particles. Warmer temperatures during addition of the CO2 tend to produce scalenohedral (rosette-shaped) PCC particles. In either case it is critical to end the reaction at an optimum pH where the milk of lime has been effectively converted to CaCO3, and before the concentration of CO2 becomes high enough to acidify the suspension and cause some of it to redissolve. In cases where the PCC is continuously agitated and stored no more than a few days, it may be unnecessary to add more than a trace of such anionic dispersants as polyphosphates. On-site PCC usually has a weak cationic colloidal charge. By contrast, dried PCC can be similar to most ground CaCO3 products in having a negative charge, depending on whether dispersants have been used.
Function: Filling of paper, especially in cases requiring high brightness. Rosette-type PCC is used in applications requiring opacity improvements and maintenance of caliper at specified levels of weight and smoothness. Rhombohedral PCC products have effects more similar to those of ground CaCO3 products.
Strategies for Use: Many of the considerations for use of PCC filler are the same as those that apply in the case of other forms of calcium carbonate. The fact that PCC tends to be weakly cationic, and the other forms of the mineral tend to be anionic, can have a subtle effect on retention, but usually there is no significant problem. Most well-designed retention aid programs are affective with either type of CaCO3 filler. Because scalenohedral (rosette) PCC tends to lower the density of paper (especially before it is calendered) it can be more difficult to press water out of the paper made with that form of PCC. Sizing agents such as AKD do not form permanent bonds to the surface of PCC. When AKD and PCC are both used in the same paper machine system it is recommended to add them at widely separated points, usually with the filler added first. Evidence suggests that this practice allows colloidal material including starch to coat the surfaces of the filler particles, giving the AKD something secure to attach to. Also, the coating may keep the AKD from coming into contact with residual Ca(OH)2 in the PCC. In certain cases the weakly cationic surface of PCC helps it to be effective in the collection of tacky materials from the furnish.
Cautions: Usually there are no significant hazards associated with PCC use in the paper mill.
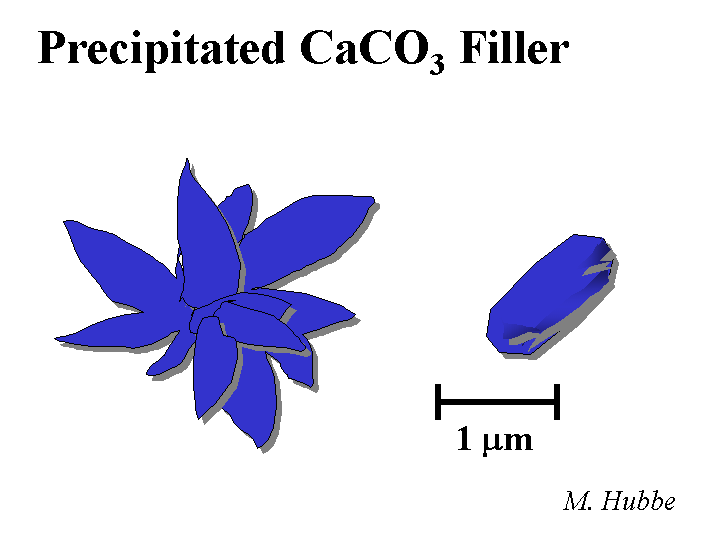
RETENTION AID
Composition: The words “retention aid” most often refer to cationic or anionic acrylamide copolymers having molecular mass values in the range of 5 to 20 million grams per mole. These are known to be effective for improving the retention of fine particles during formation of paper. However, it is worth noting that many additional or alternative materials also can be used. Since some of these additives have to be used in various combinations, one may speak of a “retention aid program.” The following sequences are used commonly: (a) a high-charge cationic polymer followed by a very-high mass cationic polymer (usually cPAM or PEI copolymer), (b) a high-charge cationic polymer followed by a very-high-mass anionic polymer (usually aPAM), (c) a very high-mass cationic polymer followed by an anionic microparticle such as colloidal silica, bentonite, or a micropolymer, or (d) options involving polyethylene oxide (PEO).
Function: To maintain adequate efficiency, drainage, and cleanliness of a paper machine; to achieve adequate Z-directional uniformity; to retain additives, including fillers.
Strategies for Use: Retention aids can have a profound effect on the efficiency of the process and on the quality of the product. For instance, an overdose of retention aid is likely to produce a highly flocced sheet that is unacceptable to the customer. So the most general rule is to add only the amount of retention aid that is needed to optimize runnability and avoid excessive problems of two-sidedness of the sheet structure. Relatively high retention is desirable, however, when using ASA size or when using rosin emulsion size under neutral or alkaline pH, since those additives will tend to gradually decompose if they are not retained in the first pass. Additional strategies are mentioned under the headings for several of the additives that are often used in retention aid programs.
Cautions: Consult the MSDS for each additive. Be aware that many different patents exist that can affect one’s right to use different combinations of additives.
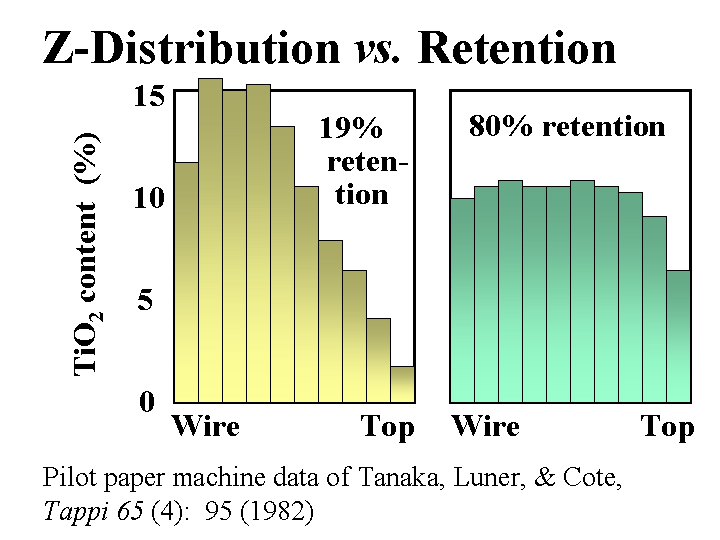
ROSIN EMULSION SIZE
Composition: The word “rosin” refers to a series of chemicals isolated from the “tall oil” that is produced during kraft pulping of softwood species. One of the major components of softwood rosin is abietic acid, a partially unsaturated compound with three fused six-membered rings and one carboxyl group. It is common to treat the native rosin with fumaric acid, converting at least some of the abietic acid and related compounds to tricarboxylic species called “fortified rosin.” Rosin acids are tacky solids at room temperature. Something has to be done before they can be added effectively to a paper machine. One of these things is to form an emulsion. The supplier of the rosin accomplishes this by heating the rosin above its melting point and applying hydrodynamic shear as it is poured into a hot aqueous solution in the presence of a stabilizer. The pH may be adjusted so that at least a small amount of rosin on the surface of the droplets is saponified to the carboxylate form. That solution may contain casein or various cationic polyelectrolytes as stabilizers to help the emulsion from coalescing into larger particles.
Function: To resist penetration of aqueous liquids into paper
Strategies for Use: It is most common to use rosin emulsions under mildly acidic conditions, e.g. between pH value of about 4.5 and 6. These are conditions that favor very high retention of alum or PAC on the fibers. Rosin emulsions are most often used in conjunction with a “reverse addition” strategy for alum and rosin. In other words, the soluble aluminum-containing product is added first. This order favors complexation of any dissociated carboxyl groups with aluminum species, an interaction that is favorable to sizing. At the same time, this practice tends to minimize the opportunity for the rosin to react with calcium. Usually it is recommended to have the addition points relatively close in time, but with good mixing of the furnish between the two addition points. There can be exceptions when attempting to reach very high pH values; in such cases it is sometimes recommended to pre-mix the rosin emulsion and a solution of PAC or alum with specialized equipment before it encounters the furnish. High first-pass retention is recommended to minimize the gradual conversion of the free-acid form of rosin to its dissociated (saponified) state, which is more stable under the conditions of use (pH > 4.5). It is worth noting that somewhat different usage strategies apply when one is using rosin soap as a sizing agent. It is also worth noting that the material from rosin emulsion droplets mostly does not interact with alum until the paper is dried. Despite this, it is still important to use an aluminum source (alum or PAC) to help retain the rosin emulsion and so that the rosin molecules anchor and orient themselves on the surface of the fibers when they encounter the high temperatures of the dryers.
Cautions: Rosin emulsion does not pose any particular dangers. Consult the MSDS and follow the supplier’s recommendations.
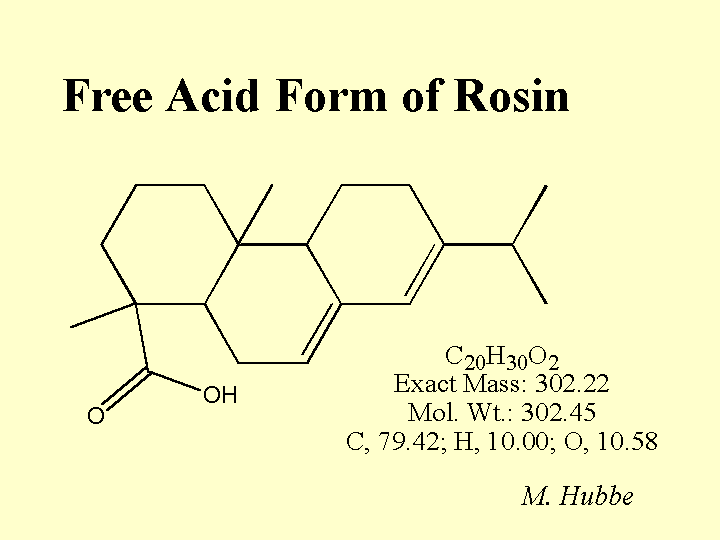
SIZING AGENTS (internal)
Composition: Even when one qualifies the term “sizing agent” with the word “internal,” it still can refer to several different materials. Some of these are discussed in greater detail elsewhere in this series. To perform their function in resisting penetration of water or other liquids into paper, sizing agents all contain some type of low-energy chemical group. These may include fatty acid “tails,” typically in the range of 14 to 22 carbons in length. Alternatively, these may consist of fused hydrocarbon rings, as in the case of rosin products. Every successful sizing agent contains some handle by which the molecules can be retained, anchored and oriented. In the case of rosin products, this anchoring point consists or one or more carboxyl group per molecule. In the case of ASA or AKD it consists of a reactive ring that is theoretically capable of forming an ester bond with hydroxyl species at the surface of paper. The degree to which such reactions actually take place under the conditions of papermaking has been a matter of debate and experimentation.
Function: To increase the resistance to penetration of paper by water or related liquids
Strategies for Use: More specific guidelines are provided in the discussions of such sizing agents as rosin soap size, rosin emulsion size, alkenylsuccinic anhydride (ASA), and alklylketene dimer (AKD). The general strategy involves such steps as (a) making sure that the size is prepared in a form that will distribute very well with the furnish, (b) mixing it with the furnish at a point where adverse interactions with the furnish are minimized, (c) using the best sequence and ratio of alum or PAC in the case of rosin sizes, (d) retaining it well, and (e) possibly adjusting the conditions of the production environment to promote more rapid cure.
Cautions: Consult the MSDS of each chemical product.
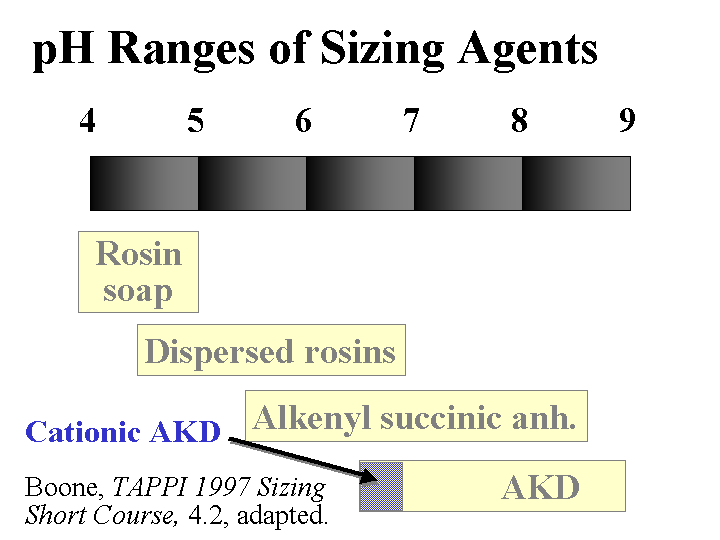
SOFTWOOD
Composition: Softwood fibers (tracheids) come from needle-bearing, conifer trees. The main chemical components of softwood kraft tracheids – cellulose and hemicellulose – are polymers of glucose, with each unit connected by a beta-1-4-glycosidic linkage. These molecules are arranged in a series of layers having different angles relative to the fiber direction. The thickest of these layers (the S2 sublayer) has a fibril angle almost lined up with the fiber, and this accounts for the high dimensional stability of both fibers and wood in the length direction. The cellulose polymers are partly fused together in microscopic crystalline domains, and this accounts for the insolubility of the wood fibers. Two main pulping methods, kraft and mechanical, are used to liberate the fibers. The kraft process dissolves a natural phenolic resin called lignin from between the fibers. In mechanical pulping the lignin is left in place. For example, in thermomechanical pulping (TMP), wood chips are passed between rotating plates having raised bars at elevated temperature and pressure. The heating softens the lignin and makes it possible to separate the fibers in an intact state, obtaining a yield of over 90% of the original solids from the wood. Softwood fibers from commonly used papermaking species such as pine, spruce, alpine fir, and Douglas fir have tracheid fibers approximately 3 mm long and 20 to 30 micrometers thick. Especially after kraft pulping the refining process tends to delaminate the cell wall, allowing the fiber to collapse into a ribbon, greatly increasing the fibers’ ability to bond to each other.
Function: Softwood fibers (tracheids) confer high tearing strength, ability to withstand multiple folding, and a range of other strength-related properties.
Strategies for Use: Softwood kraft pulp is the main component of linerboard for corrugated containers and for bag grades. Bleached softwood kraft is a key, but usually minor, component of printing papers and many other products that can benefit from the reinforcing ability of the longer fibers. Softwood chemithermomechanical pulp (CTMP) is useful in products that can benefit from decreased density. One of the key considerations in the use of softwood pulps is how to achieve a satisfactory formation uniformity. The ratio of length to width (aspect ratio) of softwood fibers is often as high as 100. That means that the fibers have a strong tendency to clump together when suspended in water. In theory one would have to reduce the solids level to less than about 0.01% to give every fiber room to rotate without colliding into an adjacent fiber. Thus far the paper industry has not been able to afford the extremely high pumping requirements that would be needed, in theory, to achieve such levels of dilution. Rather, it is accepted that there will be some level of nonuniformity due to fiber flocculation. Hydrodynamic shear within the paper machine system itself both causes and breaks up fiber flocs. The use of retention aids may greatly increase the nonuniformity of paper when considering areas of 1 cm2 to 100 cm2. The adverse effect of retention aids on formation can be minimized by using a lower dosage or moving the addition point before the pressure screens. Softwood kraft pulps generally can benefit from a higher level of refining, compared to hardwood pulps.
Cautions: There are no special hazards associated with softwood pulps.
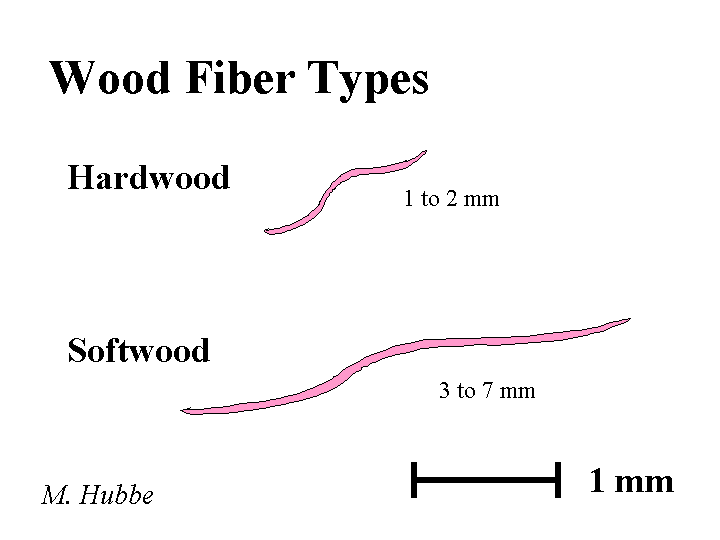
STICKIES
Composition: Stickies are one of the things you get when you recycle mixed waste paper. Actually, stickies are many things. A key component is likely to be polyvinylacetate (PVAc), a material that is widely used for self-stick labels. Stickies encountered in a mill using recycled paper are likely also to contain wood pitch, oils, sizing agents, antifoam, etc. It is the nature of stickies to be highly deformable, somewhat water-hating, and, well, “sticky.”
Function: Stickies can hurt product quality when they appear as spots or when they cause pick-outs. They can hurt paper machine efficiency due to the downtime required to clean the wet-press section or other parts of the paper machine where they decide to accumulate.
Strategies for Use: The best strategy is prevention – get it out of the furnish before the paper machine system. Sorting of waste paper is the most effective strategy, but it can be very expensive. The next line of defense is to remove stickies by screening operations. Screening of stickies often is made more difficult after pulping because the particles are broken down. Even in cases where the stickies remain intact, they still can extrude themselves through the narrow slots of a pressure screen. The fact that stickies usually have a density similar to water means that hydrocyclone cleaning is unlikely to be effective as a separation tool. However, the water-hating nature of some stickies can help promote their removal during a froth-floatation stage of a de-inking process. Some strategies to consider on the paper machine include maintenance of good retention, use of talc, continuous chemical cleaning of the wet-press felts, and use of doctor blades to keep dryer can surfaces clean.
Cautions: Solvents used for cleaning of tacky materials from papermaking equipment may be hazardous. Safe cleaning procedures should be followed.
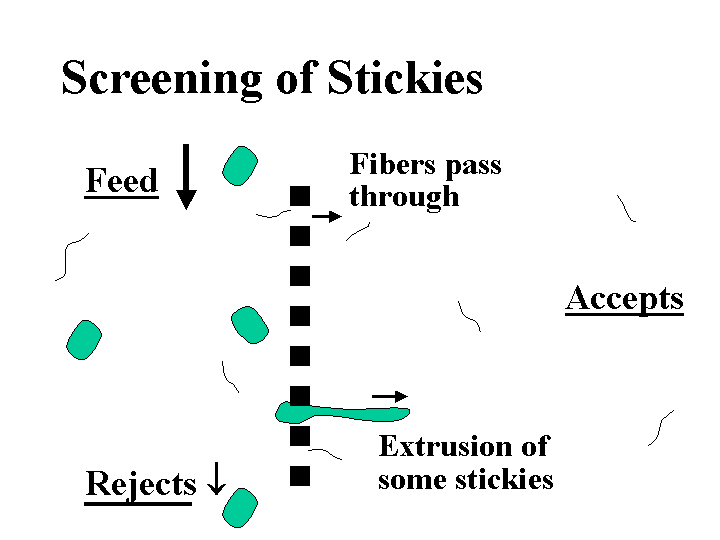
STARCH
Composition: Like cellulose, starch is comprised of glucose units linked together by oxygen bridges called glycosides. The main difference is the orientation of the molecules in that linkage (alpha rather than beta). This subtle difference means that starch is more readily soluble in water and more easily digested by bacteria and other living things. Papermakers encounter mainly two forms of starch. The starch added to the wet end is mainly cationic starch. Such starch usually has been derivatized with a quaternary ammonium compound. The degree of substitution is usually about 0.02 to 0.03 on the basis of glucose units (0.2 to 0.35% nitrogen). Because the cationization reaction is carried out with a partially swollen slurry of starch granules, the distribution of cationic groups can be expected to be very nonuniform. Papermakers also may add amphoteric starches that also contain phosphate groups, in addition to the cationic quaternary amine groups. In addition to the wet-end starches, it is very likely that a major part of the starch in a wet-end system comes from recycled broke from the size press, after a coating operation, or from waste paper. Starch from these sources is likely to be uncharged pearl starch or oxidized starch that has been partly degraded to adjust its viscosity to make it suitable for surface applications.
Function: Starch contributes to the stiffness and bonding within a sheet of paper. Cationic starch is added to improve internal bond, tensile strength, and as part of certain retention and drainage programs. It also is widely used for the preparation of sizing agents such as alkenylsuccinic anhydride (ASA).
Strategies for Use: The main thing to bear in mind when using wet-end starch is the fact that each papermaking furnish has a limitation on its ability to retain that starch efficiently. In some cases the critical factor is the surface area of the furnish. In other cases the critical factor is the limited amount of anionic colloidal charge at the fiber surfaces. In yet other cases there is competition between cationic starch and other materials such as wet-strength agents for adsorption sites. As a rule of thumb, papermakers usually are well advised to add about 10 lb/ton of cationic starch and then gradually optimize the system from that point. Addition of colloidal silica downstream of the cationic starch (Eka Nobel technology) can help drainage and retention, and it even can help achieve higher dry-strength. Dewatering aids can sometimes produce a dry-strength improvement because the easier removal of water may permit higher pressures to be applied in compaction of the fibers in the wet press. By contrast, cationic starch by itself is expected to contribute to bonding strength by increasing the effective area of bonding between the fibers.
Cautions: Spilled starch can be slippery. Starch make-down areas also may be subject to bacteria growth if not treated.
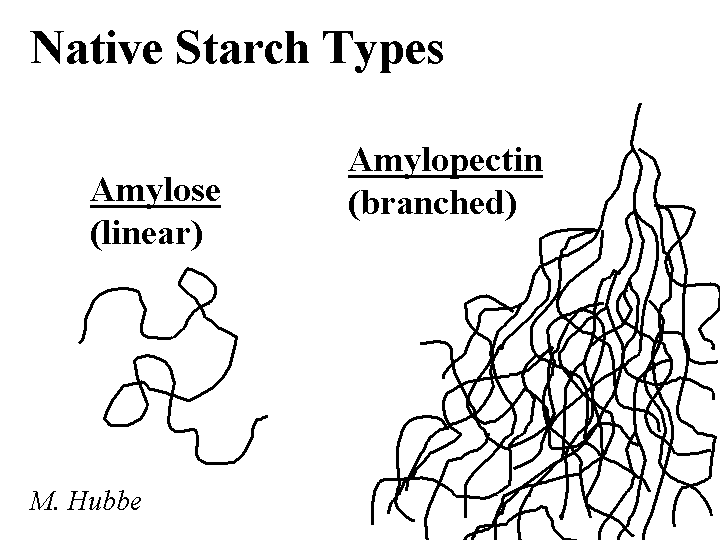
SURFACE SIZE ADDITIVES
Composition: The most commonly used surface size additives (in addition to starch) are styrene maleic anhydride (SMA) copolymers, styrene acrylates (SA), alkylated urethanes, and several latex products that include some of the same chemical functionalities. Many of these products are formulated with a strong anionic charge. Usually they are delivered as viscous solutions comprised of the sodium or ammonium salts of the corresponding poly-carboxylic acids.
Function: These materials are not added to the wet end, but they have a close relation and interaction with wet-end additives. Their purpose is usually at least partly the same as that of wet-end sizing agents –reduction in the tendency of water to penetrate into paper. In addition, many of these materials can increase the surface strength of paper and decrease air permeability to a greater degree than a film of starch alone at the paper surface.
Strategies for Use: Briefly stated, successful use of hydrophobic size-press additives can be sensitive to pH. The pH has to be high enough to keep the additives dissolved and to minimize foaming. Defoamer use has to be carefully optimized, since defoamers can work against the desired sizing effect. The performance of many surface size additives can be greatly improved if the paper already contains enough internal sizing agents to keep the size-press starch film near to the paper surface. There has been no documentation of the effects of surface size additives recycled back to the wet end with broke.
Cautions: Size press starch is very hot and may cause burns.
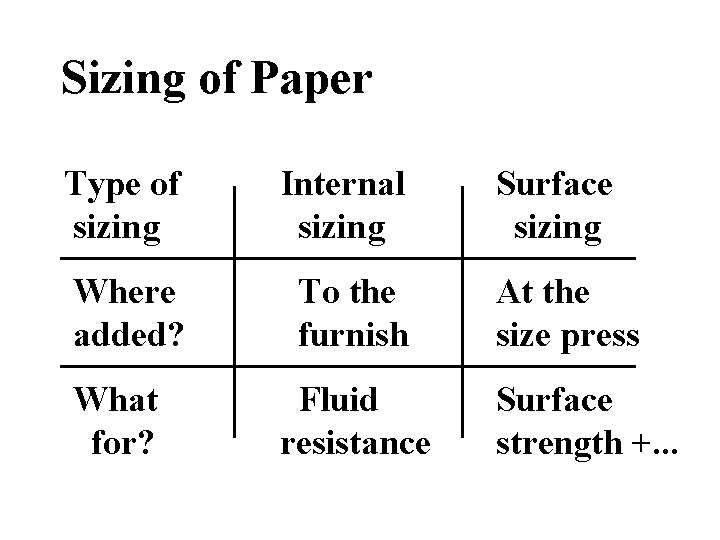
TALC (for pitch control and as a filler)
Composition: The chemical formula of pure talc is magnesium silicate, Mg3SiO4O10(OH)2. Talc is a soft mineral (hardness = 1) with a relatively water-hating surface. These two attributes, softness and hydrophobicity, are easy to observe when one pinches some talcum powder and then releases it onto the surface of water in a sink.
Function: Reduce the tackiness of pitch-like materials or stickies so that they have less tendency to form agglomerates or deposit onto papermaking equipment or create spots in the product. Also, the function of talc is to reduce tackiness of materials that already have deposited, so that further accumulation of tacky materials on those surfaces is slowed.
Strategies for Use: Some of the key rules of thumb for talc are (a) disperse it well, (b) add it the those process streams that are giving the biggest problems, (c) add it early enough to the process with enough agitation, and (d) add enough. If too little talc is used, the talc is merely incorporated into deposits and agglomerates of tacky materials. It makes sense also to use an effective retention aid program in order to retain the talc (and any attached tacky materials), purging the problem from the wet-end system. Similar considerations apply to the use of organic detackifiers. In either case, the best place to start in dealing with deposit problems is chemical analysis. Several major papermaking research groups (including this one) have equipment for lab-scale simulation of pitch and stickies problems.
Cautions: Talc is potential source of dust. Consult MSDS information for each detackifier. Lock-out procedures and air quality checks are required when tanks and headboxes must be entered to inspect deposits.
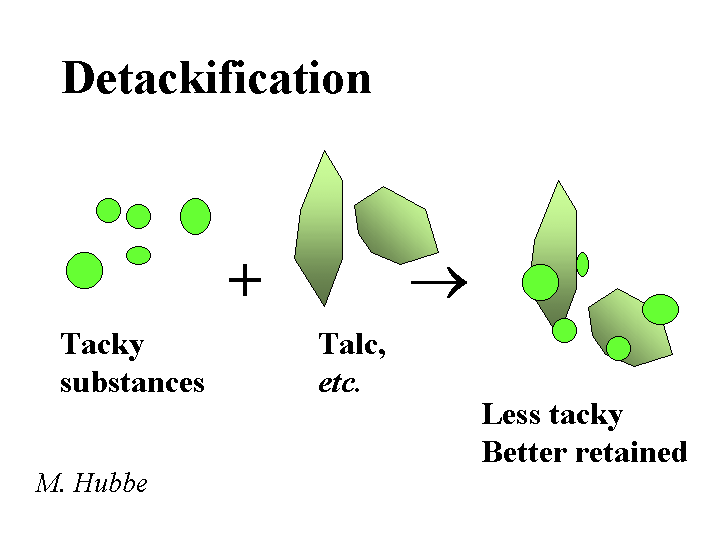
TITANIUM DIOXIDE
Composition: TiO2. Pigmentary titanium dioxide, as used by the paper industry, usually consists of semi-spherical particles having diameters all very close to the range 0.25 to 0.4 micrometers. Most of this is delivered to paper mills in the form of aqueous slurries having solids levels near to 70%. This may seem to be an impractically high number until one considers the fact that the mineral is much denser than water, so the volume fraction is much lower than the mass fraction. Dispersants used in stabilizing the aqueous slurries can contribute a very high negative colloidal charge to the system. In addition, the surface or the bulk phase of TiO2 products is often treated so that it contains a minor amount of such materials as aluminum, silicon, or phosphorous, and such treatments also affect the colloidal charge.
Function: To increase the opacity of paper products, the brightness of white-top linerboard and similar products, and brightness of some paper products.
Strategies for Use: The important thing to remember about titanium dioxide particles is that they increase opacity by scattering light. Their ability to scatter light depends on their being present in the sheet as separate particles, not clumps. This means that the TiO2 product should be well dispersed before it is added. Premature mixing of titanium dioxide slurry with alum or other cationic materials should be avoided in order to minimize self-agglomeration of the pigment. Good optical efficiency usually can be achieved by first adding the pigment at a place where it becomes well mixed with the furnish, and then adding a retention aid. Even better optical efficiency can be achieved in some cases if enough highly charged cationic polymer is added before the TiO2 to create cationic sites on the surfaces of fibers and fines. The amount has to be optimized, since an excess of cationic polymer in the solution merely will agglomerate the TiO2 to itself. Some of the negative features of titanium dioxide are (a) relatively high abrasiveness, and (b) absorption of ultraviolet light, reducing the effectiveness of fluorescent whitening agents. Though TiO2 is often used to meet brightness goals, this approach tends to be expensive relative to other options such as increased bleaching and use of calcium carbonate filler, etc.
Cautions: Titanium dioxide can create quite a mess, but, with effort, it can be cleaned up. Even trace amounts of TiO2 in effluent water will tend to make it turbid.
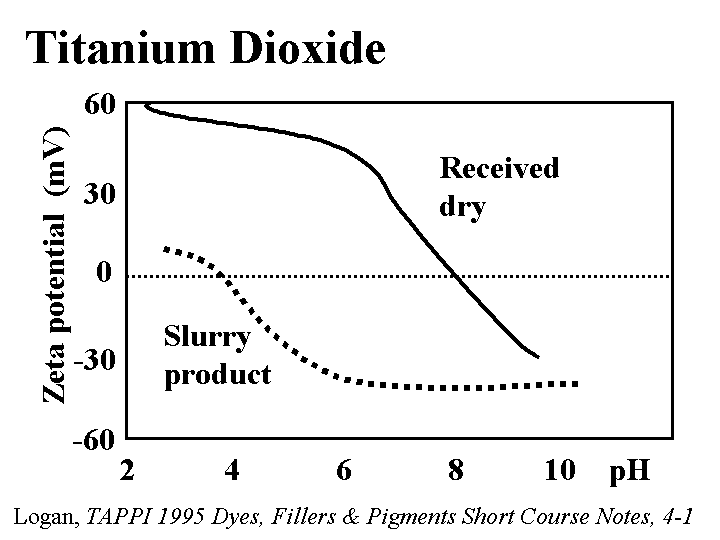
TONER (xerographic printing)
Composition: By far the major pigment component used in xerographic toner production is carbon black. But from a papermaker’s standpoint the behavior of toners is most strongly affected by the binders used in their formulation. The chemistry of these binders is diverse and ever-changing as new laser printers and photocopiers are introduced. The one common feature is that the toners have to be able to melt and fuse onto the rough surfaces of paper. Xerographic printing can cause the toner to be partly interlocked with the roughness of fiber surfaces. Most, but not all of the toner can be released by the high levels of hydrodynamic shear during pulping and dispersing operations when waste paper is de-inked. Most of the detached toner particles are then removed either by washing, floatation, or agglomeration into particles large enough to be screened out of the mixture. The other notable attributes of most toner particles are (a) somewhat hydrophobic character, and (b) usually anionic charge.
Function: For xerographic printing. During papermaking, toners present in the furnish (from wastepaper) tend to reduce brightness of the product, create spots, and possibly deposit on papermaking equipment. The thermoplastic nature of toner binders means that it is likely that there will be a deposit issue in the early dryers.
Strategies for Use: This is another case where the best solution is to remove toner particles before they reach the wet end. Dryer-can adhesion problems are likely to become less severe if the first-pass retention is kept high, keeping the fine material on the fibers rather than in the residual water still in the sheet. The idea is that free water that flashes out from the sheet in the dryer section will deposit any thermoplastic particles not anchored onto the fiber surfaces. The down-side of an efficient retention aid system is that it can slightly decrease the brightness of the sheet by improving retention of toner.
Cautions: Dryer-can surfaces can be extremely hot. Collection of deposit samples from such surfaces can be hazardous.
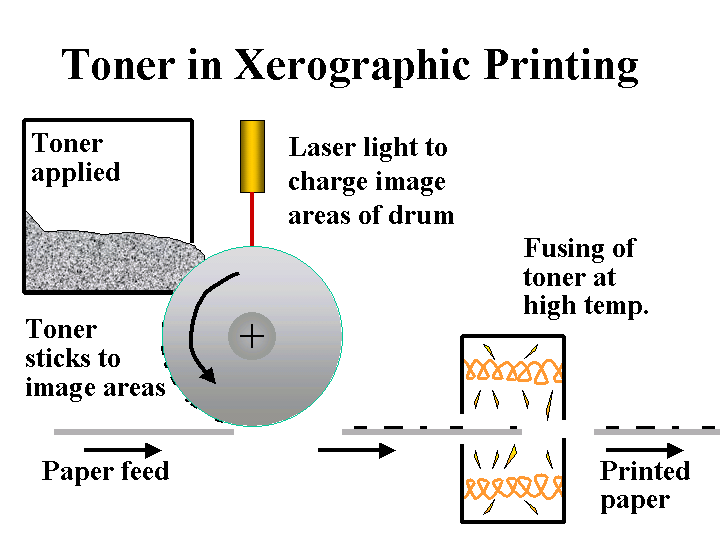
UNBLEACHED KRAFT PULP
Composition: Kraft pulp is what you get after you place a chip of wood in a pressurized vessel in the presence of hot caustic soda and sodium sulfide. The cooking process attacks and eventually dissolves the phenolic material called lignin that glues the fibers to each other in the wood. The word “Kraft” means “strong” in the language of its origin. German manufactures of pulp discovered that addition of the sulfur to a “soda cook” improved the selectivity of the process – dissolving the lignin with less damage to the cellulose. The fibers initially liberated by kraft pulping usually are brown, similar to a typical grocery bag (paper type) or corrugated box. Kraft pulps tend to be more flexible than fibers produced by purely mechanical refining of pulp. The superior bonding potential of kraft pulps becomes evident when they are refined sufficiently to allow collapse of the lumen, delamination of the cell wall, and partial fibrillation of the surfaces. Kraft fibers tend to be more porous, compared to mechanical fibers. Slit-like pores having widths in the range of 5 to 20 nm appear to occupy the space formerly occupied by lignin. Each time that paper is dried, many of these pores appear to close irreversibly. Under ordinary condition of repulping, the fibers do not regain all of their original ability to swell with water and form inter-fiber bonds as strong as those from refined, never-dried pulp..
Function: Main structural element in many paper grades, including corrugated boxes, bags, and filters.
Strategies for Use: One of the keys to successful use of kraft pulps is optimization and uniformity of refining conditions. Refining usually is needed in order to meet bonding strength objectives. However, too much refining causes excessive damage to individual fibers, yielding paper that may be too thin and too easy to tear. In such cases dry-strength additives such as cationic starch may be used in order to meet bonding strength requirements with less loss of bulk and tear strength. Uniform refining can be achieved by a combination of approaches. These include control of the consistencies and flows to a refiner, control of the amount of energy expended per dry-mass of pulp, and on-line freeness control (usually not available). Higher pH conditions (in the range up to about 8) favor more rapid refining with less damage to the fibers, especially in the case of high-yield kraft pulps. Hardwood and softwood can be refined separately, then recombined, since the softwood usually can benefit from more severe or prolonged mechanical treatment. In general, a kraft pulp blend that is rich in softwood fibers (average length about 3 mm) is easy to run on a paper machine and the paper is tough. A kraft pulp blend rich in hardwood fiber is less forgiving of non-ideal tensions in open draws on the paper machine and the product is more easily torn. Unbleached softwood kraft pulp contains wood rosin, making it practical to achieve a moderate degree of water resistance by addition of alum to the furnish.
Cautions: Kraft fibers and slurries of these fibers are usually non-hazardous if one follows usual safe practices.
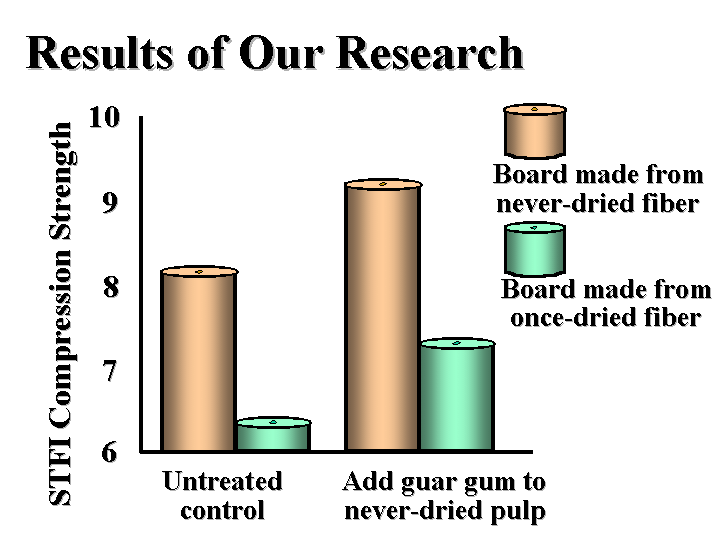
Results of tests carried out by Min Zhang at NC State University. Addition of guar gum to never-dried fibers increased the compression strength during primary sheetmaking and recycled sheetmaking.
WET-STRENGTH RESIN
Composition: This polyelectrolyte is formed by the reaction between adipic acid and diethylene triamine, and subsequent derivatization of the resulting copolymer with epichlorohydrin. Readers with a strong background in chemistry will recognize that the initial polymerization during the production of PAAE creates a chain very similar to that of Nylon. What’s different is that the chain contains secondary amine groups. Some of these react with the epichlorohydrin, and some of them remain in the product, helping to make it highly cationic and soluble under the conditions of use. The reaction with epichlorohydrin creates highly reactive aminochlorohydrin and azetidinium chloride (ring) groups along the chain. These groups appear to be capable of reacting with either the carboxyl groups at a cellulose surface, or amine groups on adjacent molecules of the additive during drying of paper. The groups added to the molecular backbone by reaction with epichlorohydrin are also highly cationic.
Function: Wet-strength agent especially well suited to neutral and alkaline papermaking; also sometimes used in formulation of cationic rosin size emulsions
Strategies for Use: The first consideration is shelf life. Storage for too long or too warm will cause PAAE to react with itself sufficiently that it looses its activity. Another thing to recognize from the beginning is that long exposure to hot papermaking furnish will reduce the performance of PAAE resin as a wet-strength agent. These factors would suggest that the best addition point would be close to the headbox of the paper machine. This would be true except for one other consideration; strength agents often are most effective when they are present on the fibers, rather than on fines. Since fines have surface areas at least three times that of fibers (on a mass basis), the fines are likely to adsorb a disproportionate amount of the additive. This effect is further amplified if one adds the resin in the thin stock – the part of the paper machine system where the relative concentration of fines in the furnish is at its highest. For that reason, strength resins often are added to the thick stock just before it is diluted at a fan pump. The performance of PAAE resin is strongly affected be the anionicity of the fiber surfaces. For this reason it can be easier to develop high levels of wet strength when using high-yield fibers. Another strategy to increase the anionic character of the fibers is to add the PAAE under alkaline papermaking conditions where the carboxyl groups on the fibers are mostly dissociated.
Cautions: Epichlorohydrin monomer may be hazardous, and suppliers of PAAE products have been able to reduce its presence to very low levels. Consult the MSDS.
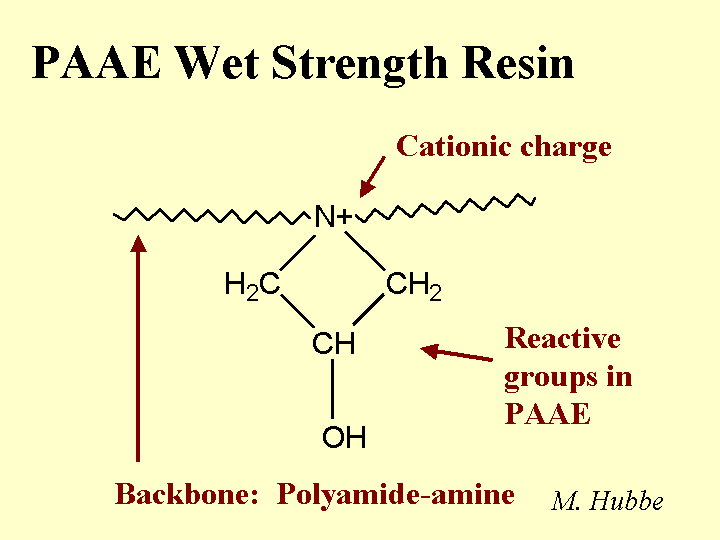
Structure of an azetidinium group, a source of cationic charge and reactivity on PAAE wet-strength resins
WHITE PITCH
Composition: The major component of “white pitch” sometimes observed in paper machine systems is latex binder from recycled coated broke. This can include styrene-butadiene resin (SBR), acrylic resins, and polyvinylacetate latex components. It also can contain the associated coating pigments, including clay, plastic pigments, calcium carbonate, and titanium dioxide. White pitch problems are often made more difficult due to mixing with wood resins, defoamer components, and other tacky materials in the furnish.
Function: White pitch can cause reduced papermaking efficiency due to felt filling and down-time required to clean up equipment.
Strategies for Use: It is highly recommended to do a chemical analysis. This may make it possible to determine the most likely root causes of the problem. One of the most general strategies for avoiding pitch problems is to keep the tacky materials bound to the fibers. This is one of the ideas behind a common practice of adding alum during or immediately after mechanical pulping. The alum complexes with any soaps of resin acids and fatty acids, and the presence of these complexes can serve to bind the multi-component droplets of pitch to the fiber surfaces. Addition of highly cationic polymers such as polyethyleneimine (PEI) to the process can have a similar effect. The relative turbidity of filtrate obtained from different points in the process can be used as an indication of the probable effectiveness of such treatments with cationic materials. It is very common also to add talc or another detackifying agent to coat tacky particles and minimize their tendency to form large agglomerates, deposits, or spots in the product. An effective retention aid system can help limit the filling of press felts with pitch.
Cautions: Safe procedures must be followed when collecting samples of pitch deposits. Equipment must be locked out and the air quality checked by qualified people before entering enclosed areas such as headboxes.
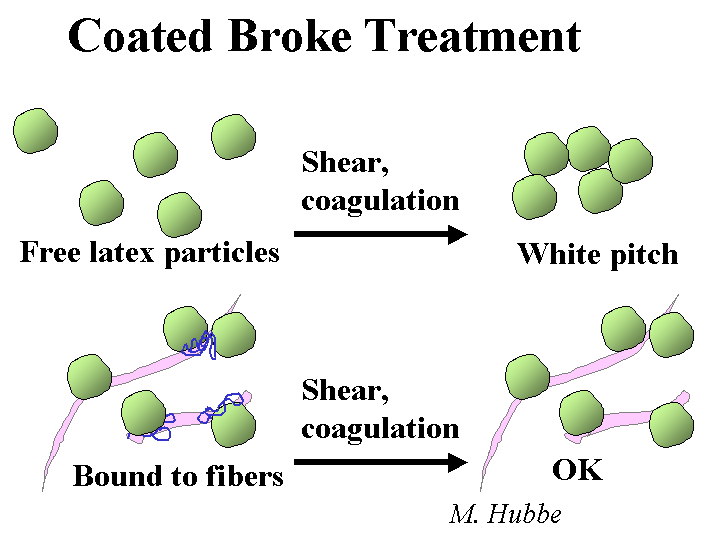